Microsoft word - 10-07013.doc
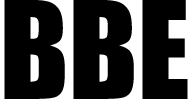
=Biotechnology and Bioprocess Engineering 2007, 12: 60-72
Enhancement of Erythropoietin Production
in Recombinant Chinese Hamster Ovary
Cells by Sodium Lactate Addition
q É=_çç= ÜçÉQI= åÇ=fâJeï å=háãNG=
1 College of life Sciences and Biotechnology, Korea University, Seoul 136-701, Korea
2 Microbiology Section, College of Pharmacy, Chung-Ang University, Seoul 156-756, Korea
3 Division of Applied Chemical Engineering and Bio Engineering, Hanyang University, Seoul 133-791, Korea
4 Department of Microbiological Engineering, Konkuk University, Seoul 143-701, Korea
Abstract The stabilization of optimum pH for cells can cause a higher erythropoietin (EPO) production rate and a good growth rate
with the prolonged culture span in recombinant Chinese hamster ovary (r-CHO) cells. Our strategy for stabilizing the opti-
mum pH in this study is to reduce the lactate production by adding sodium lactate to a culture medium. When 40 mM so-
dium lactate was added, a specific growth rate was decreased by approximately 22% as compared with the control culture.
However, the culture longevity was extended to 187 h, and more than a 2.7-fold increase in a final accumulated EPO con-
centration was obtained at 40 mM of sodium lactate. On the condition that caused the high production of EPO, a specific
glucose consumption rate and lactate production rate decreased by 23.3 and 52%, respectively. Activity of lactate dehydro-
genase (LDH) in r-CHO cells increased and catalyzed the oxidation of lactate to pyruvate, together with the reverse reaction,
at the addition of 40 mM sodium lactate. The addition of 40 mM sodium lactate caused the positive effects on a cell growth
and an EPO production in the absence of carbon dioxide gas as well as in the presence of carbon dioxide gas by reducing
the accumulation of lactate. KSBB
Keywords: erythropoietin, high productivity, lactate, CHO, lactate dehydrogenase, pH
====INTRODUCTION
Periodic replenishment of glucose and glutamine through a
fed-batch operation is commonly practiced to overcome their
Mammalian cell cultures including the culture of Chinese
exhaustion. Waste products excreted by a mammalian cell
hamster ovary cells are used extensively to produce proteins
itself have the significant effects on a cell growth and the
for a therapeutic use [1-6]. The production process of re-
production of metabolites. The major waste product is lactate,
combinant proteins depends on a high cell yield, a prolonged
which is produced mainly from glycolysis for energy acqui-
culture span, steady productivity, and consistent glycosyla-
sition and in a small amount from glutamine metabolism,
tion. The depletion of nutrients and the accumulation of cel-
and ammonium, which is excreted when amino acids are
lular waste products are two major factors limiting cell
metabolized in cells [7,8]. The concentration of lactate in
growth in mammalian cell cultures. As a result, the cell den-
mammalian cell cultures depends on the concentration of
sity in a conventional batch culture is usually low and the
glucose, a type of cell, and a mode of bioreactor operation.
culture span is short. Consequently, the amount of a product
Lactate inhibitions to mammalian cells are mainly attributed
is small, which is undesirable for the production process of
to the acidification of a medium. Namely, lactate accumula-
recombinant proteins. Both glucose and glutamine are the
tion often exceeds the buffering capacity of a medium,
major carbon and energy sources used by mammalian cells,
thereby lowering a culture pH value and inhibiting a cell
and these nutrients are often depleted during the cultivation. growth. Because mammalian cells grow rapid in vitro only
over a very narrow pH range within 0.2 to 0.4 pH units of
*Corresponding author
the optimum, cell growth rates decrease at high lactate con-
Tel: +82-2-3290-3447 Fax: +82-2-927-9028
centrations [9,10]. However, as compared to ammonium, the
e-mail: [email protected]
inhibition of cell growth by lactate occurs at relatively higher
Biotechnol. Bioprocess Eng. 61=
concentrations [11]. The toxic effects of lactate on mammal-
lactate was significantly decreased through a rational me-
ian cell cultures are well known and widely reported on a
dium design and a stoichiometric feeding of nutrients [25].
number of different cell lines [9,11-13]. For CRL-1606 hy-
In this study, our objective is to decrease the formation of
bridoma cells, lactate was found to be the only environ-
lactate by the design of an initial medium that would provide
mental parameter that significantly inhibited antifibronectin
a starting environment to achieve an optimal cell growth and
monoclonal antibody production, but the cell growth was
product production. We expected that the lactate added to a
slightly inhibited even at 40 mM lactate [14]. Stimulation of
medium could alter cell metabolism. Because it was reported
the cell growth by lactate concentrations up to 22 mM and
that cell metabolism was affected by the environmental con-
inhibition above 28 mM lactate were reported in hybridoma
ditions such as pH and the concentration of metabolites
cells (VII H-8) [15]. Miller et al. did not see any inhibition
[26,27]. We investigated whether the addition of sodium
of lactate on the cell growth over the range of lactate concen-
lactate could cause higher EPO productivity in r-CHO cell
tration considered [16]. In addition, they observed almost no
cultures, and also examined effects of the lactate addition on
change in metabolic rates when lactate concentration was
lactate production and glucose consumption. Finally, the
changed from 25 to 44 mM. Ozturk et al. reported that a
relationship between LDH activity and lactate addition was
specific growth rate of mouse hybridoma 167.4G5.3 cells
was reduced by one-half at an initial lactate concentration of
55 mM [11]. A tolerance toward lactate is specific in cell
lines [16]. A difference in the effects of lactate on different
MATERIALS AND METHODS
cell types may lie on the sensitivity of key enzymes to the
glucose metabolic pathway and the metabolic shift in re-
Cells and Their Maintenance
sponse to the adverse environment.
As previously mentioned, some cell lines such as hybri-
r-CHO cell line producing EPO used in this study was
doma are characterized with respect to the effects of lactate. provided by Dr. H. J. Hong from the Korea Research Insti-
However, less information is available on the influence of tute of Bioscience and Biotechnology, Daejeon, Korea.
lactate on the growth and productivity of Chinese hamster Minimum Essential Medium-α (M EM -α) (Gibco, USA)
ovary (CHO) cells. CHO cells are the most frequently used supplemented with 10% (v/v) fetal bovine serum (FBS)
mammalian host for biomedical products and they can ex-
(Gibco) and 20 nM methotrexate (Sigma, USA) was used for
press a wide variety of recombinant proteins. CHO cells the cultivation of r-CHO cells. No antibiotic was added to
have two significant advantages: (1) high productivity and the medium. Cells were routinely cultured on 25-cm2 T-
(2) ability to synthesize oligosaccharide chain structures flasks (Nunc, Denmark) and grown in a model MCO-175
resembling those of the natural product. In addition, CHO incubator (Sanyo, Japan) at 37oC under atmosphere contain-
cells are not apoptotic and eventually die by necrotic death ing 5% CO2 and 95% humid air. Cells in an exponential
[17]. The choice of nonapoptotic cells may, therefore, be phase were used to seed at 105 cells/mL in 6-well plates (2.5
beneficial to the production of proteins. EPO is a growth mL per well) in duplicate in media supplemented with vari-
factor that stimulates the proliferation and differentiation of ous concentrations of indicated chemicals.
erythroid precursor cells to more mature erythrocytes [18].
This is one of the first recombinant proteins to make a suc-
cessful trip from the laboratory to the clinical use. Recombi-
nant human EPO has been approved for the clinical treat-
For experiments in the addition of lactate, sodium lactate
ment of anemia associated with chronic renal failure, as well (Sigma) was dissolved in MEM-α at 400 mM, and then the
as nonrenal anemia [18,19]. Change in a pH value by the pre-determined volume of 400 mM sodium lactate was added
accumulation of lacate alters the protein processing, include-
to a culture medium to obtain the required final concentration
ing glycosylation, and the secretion [20,21]. Many studies at the start of cel cultures. In this study, culture media with 0,
have described strategies to reduce the accumulation of lac-
5, 10, 20, 40, and 80 mM sodium lactate were used. Using the
tate in mammalian cell cultures. The usual strategy is to re-
same batch of cel s, the culture with the same concentration of
place the "spent" medium with a fresh medium. However, sodium chloride, which would give roughly the same osmolar-
this strategy increases the use of serum, reduces the yield of ity as that with sodium lactate, was also set up for the purpose
a product on serum, and ultimately raises the cost of produc-
of distinguishing between lactate effect and osmolarity effect.
tion. Glacken et al. introduced a strategy, which was to feed To test the effect of osmolarity, sodium chloride (Sigma) was
glucose into the cultures at a controlled low rate [22]. Be-
added to a medium at the concentrations of 27.5, 47.5, 62.5,
cause an increase in the concentration of glucose from 0.2 to 72.5, and 87.5 mM. These concentrations of sodium chloride
10 mM reduces the flux of glucose through the pentose-
would give those osmolarities as 380, 420, 450, 470, and 500
phosphate pathway by 50%, it preferably increases the flux mOsm/kg because the measured osmolarity of culture me-
of glucose through glycolysis to lactic acid [23]. In addition, dium was found to be approximately 325 mOsm/kg. Cells
Eagle et al. demonstrated that the substitution of more cultured in the medium with normal osmolarity (325 mOsm/
slowly utilized sugars, such as fructose, galactose, and mal-
kg) were inoculated into media with varying osmolarities and
tose, results in a small amount of the accumulation of lactate cultured in 6-wel plates (Nunc). Several aliquots of fresh me-
[24]. Xie and Wang also reported that a production rate of dium were transferred to a series of conical tubes and their pH
were adjusted using sterile NaOH and HCl. An aliquot of a
containing LDH isoenzymes 1 to 5 was used as an aid in the
prepared suspension of cel s was added to these tubes to
identification of isoenzymes. After the electrophoresis, sepa-
achieve a final cell density of 105 cells/mL. Each cell suspen-
rated LDH isoenzymes were visualized by using a colorimet-
sion was pipetted in 2.5 mL into each wel of 6-well plate.
ric detection (Procedure No. 705-EP, Sigma). The stained
During the cultivation, the supernatant of cell cultures was
gel was fixed in a methanol-acetic acid solution (75 metha-
taken at regular intervals and frozen for later analysis. Cells
nol : 5 glacial acetic acid : 20 water).
were counted for obtaining a growth profile. Al experiments
were duplicated.
Lactate Dehydrogenase Activity
Lactate dehydrogenase (LDH) activity was determined by a
diagnostic kit (Sigma). There were two procedures for deter-
Concentrations of lactate and glucose in culture media
mination of LDH activity. The first procedure was based on
were measured with a YSI model 2700 Biochemistry Ana-
the oxidation of lactate to pyruvate [lactate + NAD → pyru-
lyzer (Yellow Springs Instruments, USA). Osmolarity was
vate + NADH]. LDH catalyzes the oxidation of lactate to py-
measured by a freezing point depression on an Osmomat 030
ruvate with the simultaneous reduction of NAD. Formation of
(Gonotec, Germany). To monitor an external pH, a sample
NADH results in an increase in absorbance at 340 nm. The
of 1.0 mL was taken and immediately measured by a pH
increasing rate of absorbance at 340 nm is directly propor-
meter 440 (Corning, USA). Cell viability was determined by
tional to LDH activity in a sample. To determine LDH activity,
counting in a hemacytometer under a phase contrast micro-
the supernatant of cell lysates was prepared by following the
scope using the trypan blue exclusion.
procedure used in the detection of LDH isoenzyme. Assay
reagent contained 50 mmol/L lactate, 7 mmol/L NAD, 0.05%
Bioassay for EPO-Quantitative Analysis
sodium azide, and nonreactive stabilizer and fillers (pH 8.9).
The prepared cel extract (50 μL) was added to a assay solu-
Biological activity of EPO was examined by using an
tion (1 mL, 30oC) and mixed by gentle inversion. After the
EPO-dependent cell line, human leukemia F-36E (Riken
incubation for 30 sec at 30oC, the absorbance was read at 340
Cell Bank RCB0776, Japan), that could be maintained in the
nm by a spectrophotometer (Cary 300 Bio, Varian, Australia).
presence of EPO [28]. F-36E cell density in each well of 96-
This was initial absor-bance. Continuous incubation was per-
well plate was initially fixed to 1 × 105 cells/mL. The stan-
formed and the absorbances of 30 and 60 sec following initial
dard of EPO (Boehringer Mannheim, Germany) and the su-
absorbance reading were recorded. The absorbance reading
pernatant of each sample were diluted serially with F-36E
after 60 sec was final absorbance. Subtraction of initial ab-
cell broth and 100 μL of the dilution was assayed for the
sorbance from final absorbance was calculated to obtain ‘ab-
biological activity of EPO by measuring the viable cell den-
sorbance change per minute'. LDH activity was determined as
sity of EPO-dependent cells. After incubation for 4 days, we
follows: LDH activity [U/L] = (‘absorbance change per min-
performed MTT assay to measure viability, proliferation,
ute' × total reaction mixture volume × 1,000) / (millimolar
and activation of cells [29]. One hundred microliters of the
absorptivity of NADH at 340 nm × sample volume × light-
cultured sample was incubated once more with 10 μL of 5
path). One unit of LDH activity was defined as the amount of
mg/mL 3-[4,5-dimethylthiazol-2-yl]-2,5-diphenyl tetrazolium
enzyme that would catalyze the formation of one micromole
bromide (Sigma) in 96-well plates at 37oC for 3 h. Then 100
of NADH per minute. Specific activity of LDH was expressed
μL of 0.04 M HCl in iso-propyl alcohol (Duksan, Korea)
as units per 106 cel s. LDH activity was measured by monitor-
was added to each well, and the mixture was pipetted to dis-
ing the rate at which the substrate, pyruvate, is reduced to lac-
solve the insoluble blue formazan crystals. The plate contain-
tate [pyruvate + NADH → lactate + NAD]. Since the reduc-
ing the dissolved blue formazan was read at an optical density
tion is coupled with the oxidation of reduced form of NADH,
of 570 nm. Sample was run in duplicate.
this reaction is measured in terms of the decreasing rate of
absorbance at 340 nm. Potassium phosphate buffer (2.85 mL,
Electrophoretic Fraction and Colorimetric Detection
0.1 mol/L, pH 7.5) and 50 μL cell extract were pipetted di-
of Lactate Dehydrogenase Isoenzymes
rectly into a glass vial containing 0.2 mg reduced form of
NAD. The mixture was mixed wel , then left at 25oC for 20
Cells for this assay were harvested, centrifuged (1,000
min. This was transferred from a glass vial to a square cuvet
rpm, 2 min), and washed twice in ice-cold phosphate buff-
(1-cm lightpath) capable of holding 3 mL, and 0.1 mL sodium
ered saline (PBS). After the second washing, the viable cell
pyruvate solution (22.7 mmol/L in 0.1 mol/L potassium phos-
density was determined. Intracellular components were ex-
phate buffer) was also added to that cuvet. The absorbance
tracted by ultra-sonicating the cell pellet in 0.5 mL of PBS.
was read and recorded at 340 nm at 30 sec intervals for 3 min
The obtained cell lysates were centrifuged at 13,000 × g for
using water as reference. LDH activity in this second proce-
5 min, and LDH isoenzyme present in the supernatants was
dure was determined as follows: LDH activity [U/mL] = (‘ab-
separated by adding to 1% agarose (Bethesda Research
sorbance change per minute') / (0.001 × sample volume ×
Laboratories, USA) gel and electrophoresing in 50 V for 2 h
lightpath), where: 0.001 = ‘absorbance change per minute'
using a mini gel migration system (Mupid-21, Cosmo Bio,
equivalent to 1 unit of LDH activity in 3 mL volume with 1-
Japan). An electrophoretic marker (LDH Isotrol, Sigma)
cm lightpath at 25oC.
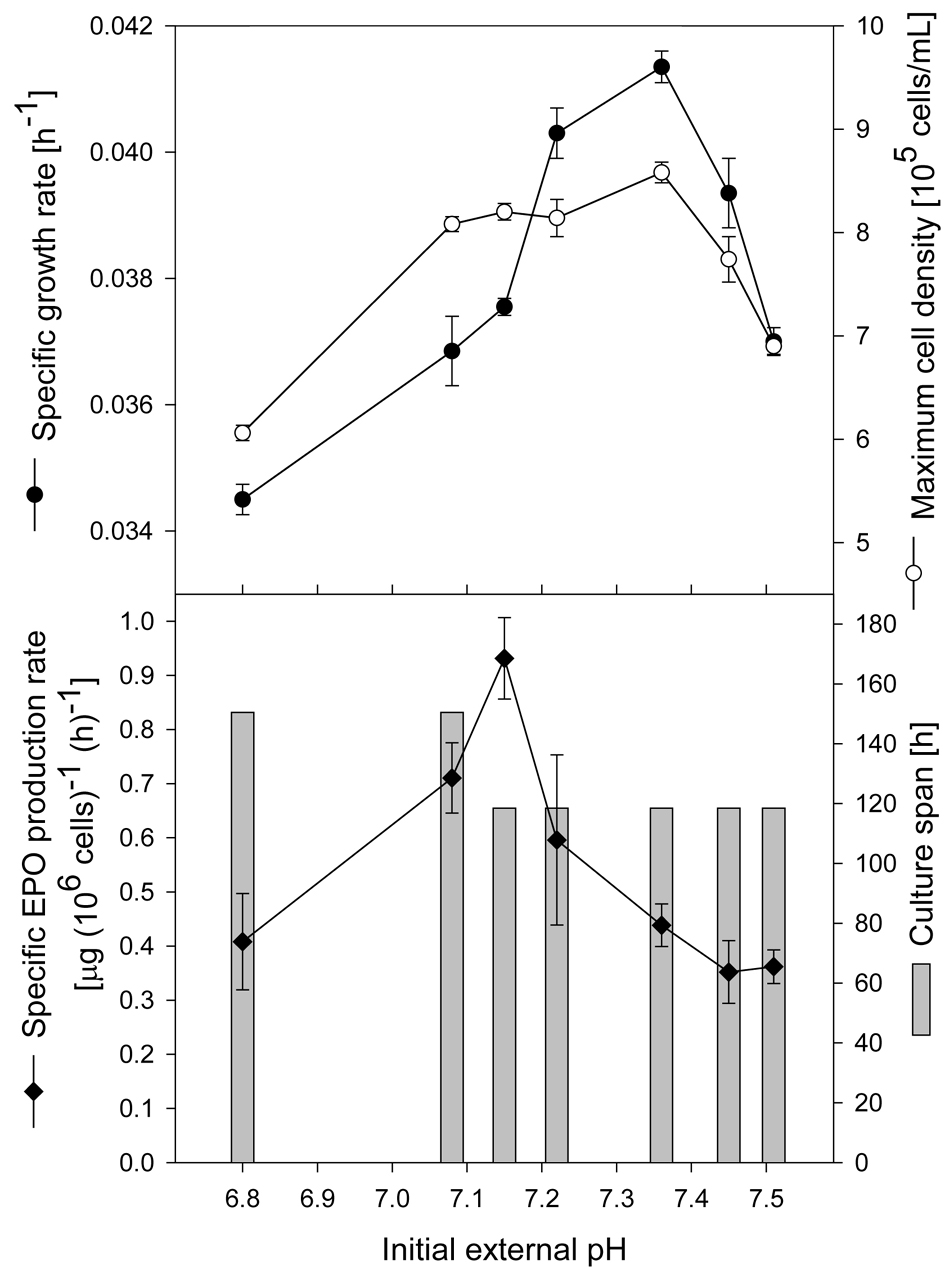
Biotechnol. Bioprocess Eng. 63=
ior was similar to that at pH 7.08. In addition, a variation of
final cell density appeared. In result, an optimal plateau for
good cell yield was obtained between pH 7.08 and 7.36, and
a maximum specific growth rate was observed at pH 7.36,
whereas the specific EPO production rate increased sharply
above pH 6.80, reaching a maximum at pH 7.15 (Fig. 1B).
Both higher EPO production rate and good cell growth were
the cause of maximum EPO production. When the initial
external pH decreased to 6.80, the specific EPO production
rate was 43.8% of that at pH 7.15. The results from Fig. 1
verify the reports that pH has a direct effect on the cells pro-
ducing EPO [20,31]. In general, external pH influences in-
tracellular pH, and the change in intracellular pH can affect
the activity of cytosolic enzymes or alter the metabolism of
cells [32]. Moreover, the pH change in an endoplasmic re-
ticulum and/or a Golgi apparatus could alter protein glycosy-
lation and secretion [21]. Gramer and Goochee also reported
that a sialidase activity in CHO cell lysates was active and
stable at pH 7 [33]. Our results, together with these reports
mentioned above, could account for the effect of initial ex-
ternal pH on the specific EPO production rate. In result, we
concluded that the pH stabilization was necessary for good
EPO production. The usual strategies for stabilizing the op-
timal pH in mammalian cell cultures are to replace the
"spent" medium with fresh medium and to titrate the pH of
Fig. 1. Effect of initial external pH on cell growth (A) and EPO
culture media with alkaline. However, the one increases se-
production (B) of r-CHO cells in MEM-α. Cells were in-
rum usage and reduces the yield of product on serum, thus
oculated at 1 × 105 cells/mL and grown in 6-well plates
increasing production costs, while the other increases media
osmolarity, which ultimately causes cell lysis. Like this, the
existing strategies have many problems. Therefore, in this
study, we introduced a new strategy, which was to add so-
RESULTS AND DISCUSSION
dium lactate as a culture supplement.
Inhibitory Effect of External pH on Cell Growth and
Increased EPO Production with Addition of Sodium
Protein Productivity
The major by-product of energy metabolism is lactate,
We examined effects of the added lactate on growth, me-
which arises from glycolysis. The accumulation of lactate
tabolism, and protein productivity of r-CHO cells producing
often exceeds the buffering capacity of a culture medium,
recombinant human EPO in batch cultures with sodium lac-
thereby lowering the culture pH. Subsequently, this may
tate of 0, 5, 10, 20, 40, or 80 mM because the addition of
cause undesirable effects on cell growth, since mammalian
sodium lactate was considered as a new strategy for the sta-
cells grow rapidly in vitro only over a very narrow pH range
bilization of culture pH in the present work. In our study of
within 0.2 to 0.4 pH units of the optimum. Unlike insect and
r-CHO cells, it seems that the specific growth rate gradually
primary mammalian cells, transformed mammalian cells
decreases with an increase of lactate addition concentration.
convert a large amount of glucose to lactate [30]. A previ-
From Fig. 2A, however, no significant reduction in the spe-
ously investigated CHO cell line was converted more than
cific growth rate was found up to 20 mM sodium lactate
97% of the consumed glucose into lactate. Effects of initial
addition. The specific growth rate was not decreased by ap-
external pH on cell growth and protein productivity of r-
proximately 22% until the concentration of sodium lactate
CHO cells transfected for the human EPO synthesis were
increased to 40 mM. We judged that the decreased specific
examined in batch cultures. Fig. 1A shows the growth results
growth rate in 40 mM would be profitable for EPO produc-
of r-CHO cells seeded at 105 cells per mL and grown over a
tion because of the following reason. If a desirable product
6 day-period at various pH values. Poor cell growth occurred
results from the expression of an amplified recombinant
at the extremes of tested pH. At pH 6.80, the specific growth
gene, the cellular energy required to synthesize this protein
rate decreased dramatically by the acidification of a culture
could be a substantial fraction of the total energy capacity.
medium, but the culture span was prolonged because of a
When cells grow rapidly, however, cellular energy sources
retarded nutrient depletion. When initial culture pH was 7.51,
may be diverted to the synthesis of materials necessary for
cells had a long lag time initially, but they were able to adapt
cell growth rather than EPO production. Therefore, fast
to pH 7.51 within a few days and subsequently their behav-
growth of r-CHO cells may lower EPO production. When
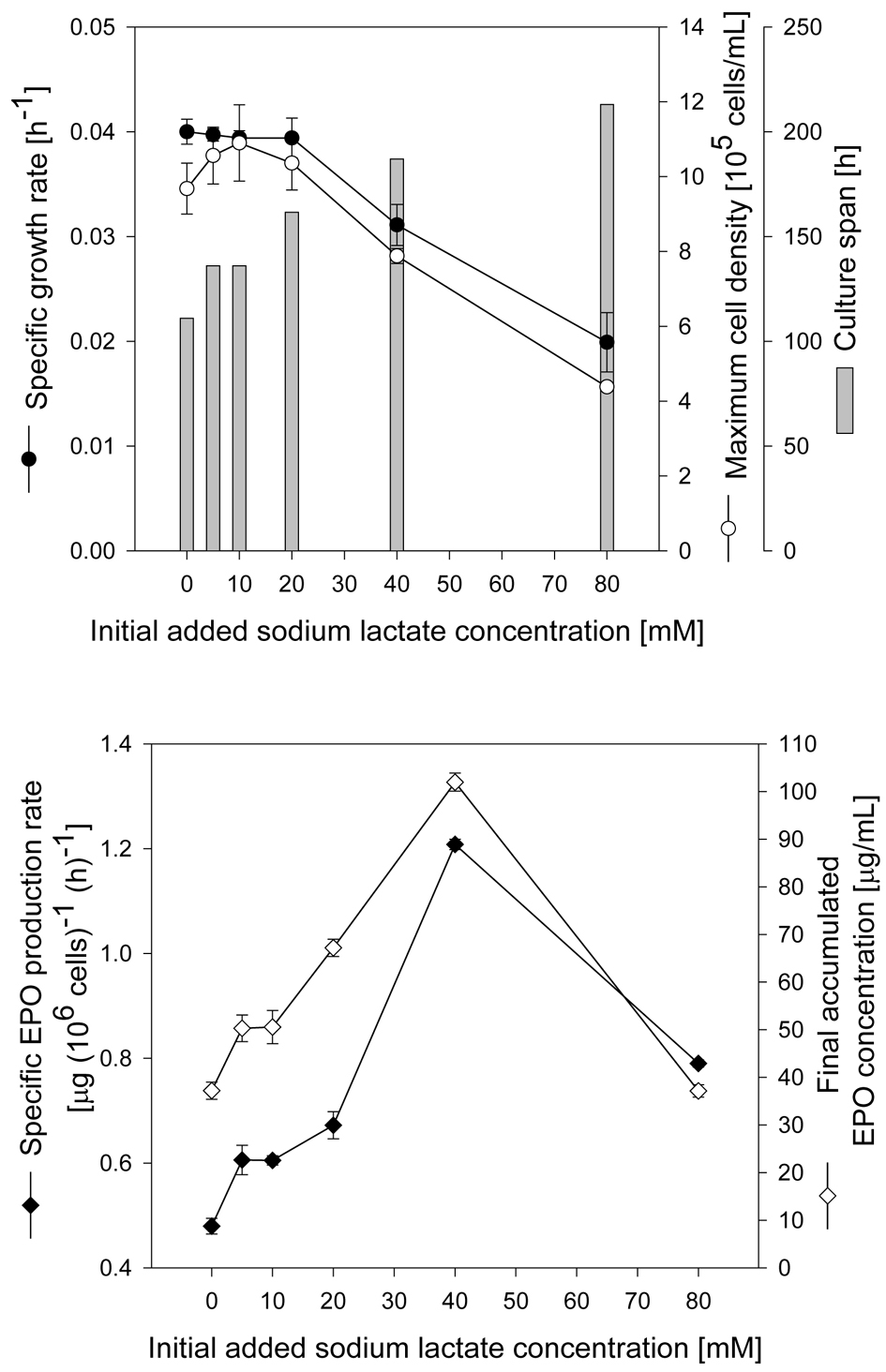
significantly to 1.21 μg/106 cells per hour in the presence of
40 mM. Consequently, more than a 2.7-fold increase in final
accumulated EPO concentration was obtained by adding 40
mM sodium lactate. The improvement of EPO production
could be acquired by prolonged cell viability and increased
specific EPO production rate. Therefore, 174% increase of
final EPO concentration observed in 40 mM lactate addition
was due to the elongated longevity of about 80 h and 152%
increase of specific EPO production rate. In addition, the
stimulation of EPO production observed here may be par-
tially the result of reduced proliferation resulting in reduction
of the cellular demand for ATP, because more than one post-
transcriptional step in EPO synthesis may be affected by
enhanced ATP availability. In conclusion, healthy cells,
longer production period, and higher specific EPO produc-
tion rate can be major factors to produce much EPO, and
these factors can be accomplished by adding sodium lactate
The entire cell metabolism could be altered in the pres-
ence of lactate, due to followed causes. The first cause is the
intracellular acidification induced by lactate. Therefore, it
has been believed that lowering lactate accumulation would
enhance cell growth and protein production, and the reduc-
tion of lactate production has been considered as one of the
important goals in mammalian cell culture technology. The
second cause is the effect of lactate in itself, especially im-
portant in the disturbance of the metabolic pathways in
which it is produced, or involved [34]. We observed only a
Fig. 2. Effect of sodium lactate concentration on cell growth (A)
marginal increase of external pH at 5 and 10 mM of the
and EPO production (B) of r-CHO cells in MEM-α. All cul-
added lactate concentrations (Fig. 3A). However, the de-
tures were initiated at an inoculum of 1 × 105 cells/mL
crease rate of pH was lowered on the condition that more
and performed in 6-well plates for 8 days. Sodium lactate
than 20 mM sodium lactate was added. This result implies
was supplemented into cultures at the concentrations in-
that the addition of sodium lactate supports the stabilization
of culture pH. The small change in external pH is profitable
for long-term cultivation as well as EPO production. Fig. 3
also shows effects of the added lactate concentrations on
the addition concentration increased further to 80 mM, much
specific rates in glucose consumption and lactate production.
depressed growth rate was observed. In this result, the in-
There was a significant decrease in specific glucose con-
hibitory concentration for a 50% decrease (IC-50) of r-CHO
sumption rate at lactate concentrations varying from 0 to 80
cells is estimated as 80 mM sodium lactate, which indicates
mM, as shown in Fig. 3B. The specific consumption rate of
that r-CHO cells are far less sensitive to lactate than other
glucose decreased by 23.2% as 40 mM sodium lactate was
cells [11]. On the contrary, a culture span was extended in
added, while the specific production rate of lactate decreased
lactate-addition cultures compared with a control culture.
by 52% (Fig. 3C). These results from Figs. 3B and 3C may
According to Fig. 2A, the culture longevity was extended for
be mainly due to lactate effect in itself. In general, continu-
between 50 and 100 h at high concentrations of lactate. In-
ous mammalian cell lines have a metabolic disadvantage of
dustrial trends toward high density cultures have required
being unable to completely oxidize glucose to CO2 and H2O,
elongating a culture span. Therefore, it is valuable to find a
although they are well accepted as reliable and safe produc-
way of elongating a culture span. From our study, we dis-
tion hosts for manufacturing pharmaglycoproteins. In other
covered that further prolonged culture span could be induced
words, most of the glucose (95 to 99.8%) is oxidized to py-
by the addition of sodium lactate.
ruvate and finally to lactate [35-37]. The reason why con-
All tested lactate concentrations except 80 mM produced
tinuous cells oxidize glucose incompletely that the enzy-
EPO far more than that in the absence of sodium lactate, as
matic connection between glycolysis and tricarboxylic acid
illustrated in Fig. 2B. For 80 mM lactate addition, the spe-
(TCA) cycle has yet to be known [38]. However, we ex-
cific EPO production rate was 1.65 times higher than that in
pected that the added lactate could lead glucose to TCA cy-
a control. However, the apparent volumetric production of
cle, because lactate could be converted into pyruvate which
EPO was similar to that in a control because the specific
could enter the TCA cycle. The yield of lactate produced
growth rate was below 0.02 h−1. The specific EPO produc-
from glucose consumed, YL/G, is an important index of gly-
tion rate of 0.48 μg/106 cells per hour in a control increased colysis. The effect of the added lactate concentrations on
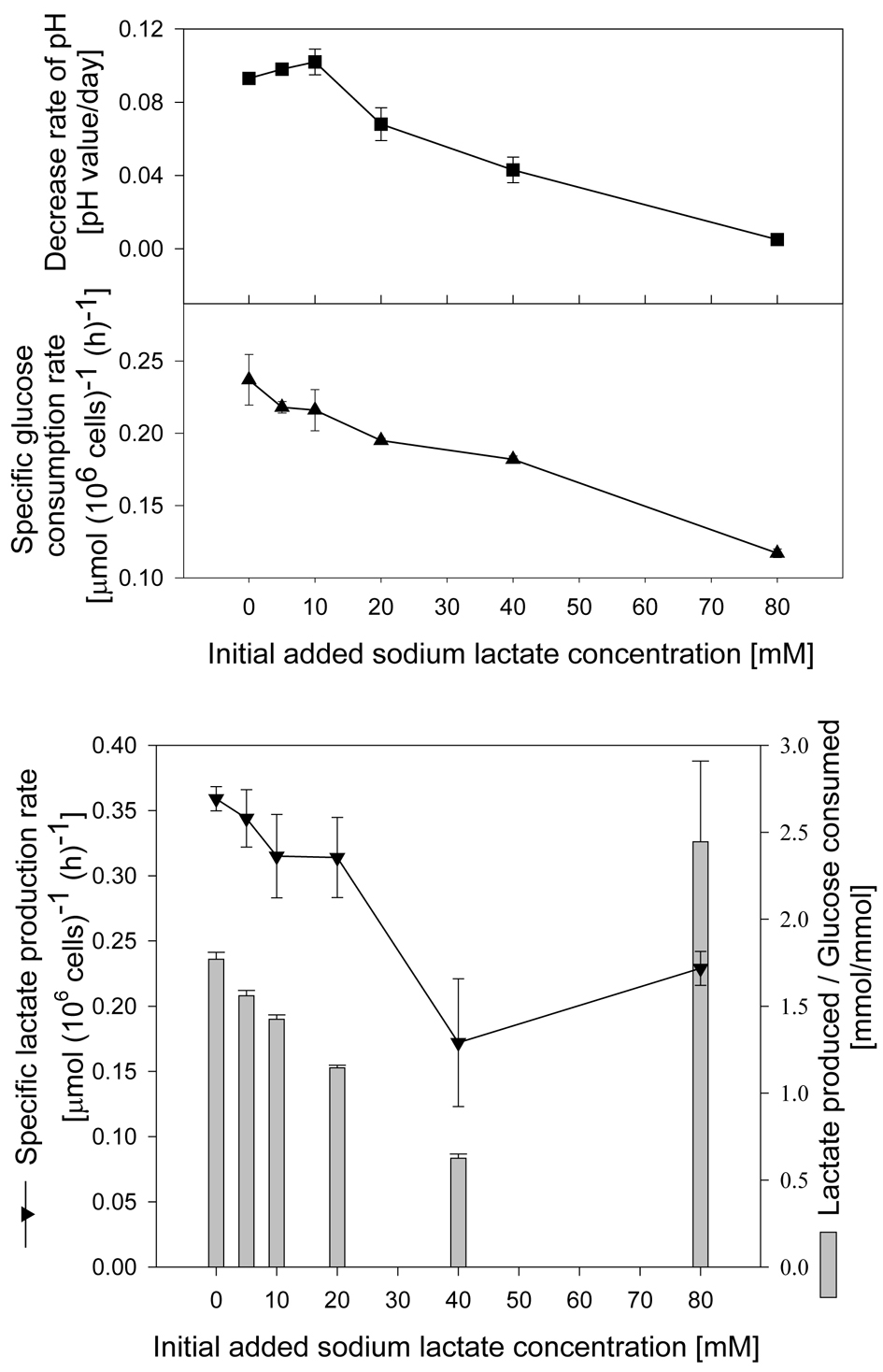
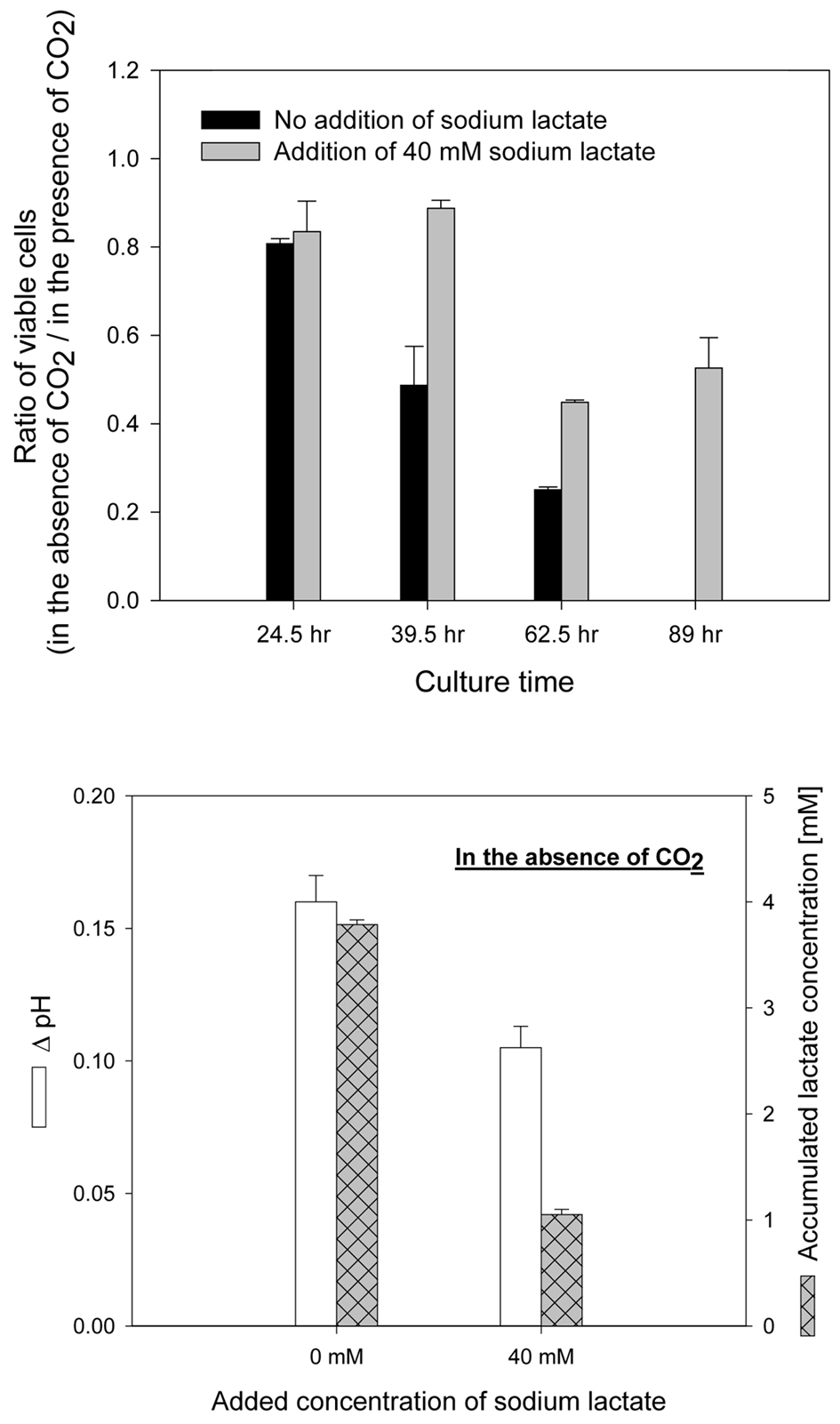
Biotechnol. Bioprocess Eng. 65=
Fig. 3. Effect of sodium lactate concentration on decrease rate
Fig. 4. Ratio of viable cells in the absence of atmospheric CO2
of pH (A), specific glucose consumption rate (B), and
to in the presence of atmospheric CO2 (A), and change of
specific lactate production rate and ratio lactate produced
pH (ΔpH) and accumulated lactate concentration of r-
to glucose consumed (C). r-CHO cells were inoculated at
CHO cells in the absence of atmospheric CO2 at the cul-
1 × 105 cells/mL and cultured in 6-well plates for 8 days.
ture time of 39.5 h (B). r-CHO cells were inoculated at 2 ×
Sodium lactate was supplemented into cultures at the
105 cells/mL in 6-well plates and cultured in MEM-α
concentrations indicated.
supplemented with 0 or 40 mM sodium lactate for 4 days.
YL/G is shown in Fig. 3C. YL/G decreased progressively as the
tralized by increasing the bicarbonate concentration (Na-
added lactate concentration increased up to 40 mM. The
3 ↔ Na+ + HCO3 ). The increased HCO3 concentration
decreased YL/G indicates that a larger proportion of con-
forces the equilibrium of CO2/bicarbonate buffer system
sumed glucose may enter the TCA cycle [39]. Judging from
toward the left until equilibrium is reached at pH 7.4. Due to
results above, the action of lactate is likely to have a pro-
the equilibrium attained between dissolved CO2 and bicar-
found effect on glycolysis, so a good amount of glucose may
bonate in this way, the pH of culture medium is maintained
enter the TCA cycle at high lactate concentrations. In result,
to the proper physiological level in buffer capacity. The ab-
an increase in energy efficiency may occur. However, the
sence of atmospheric CO2 allows the equilibrium of CO2/
elevated energy is not likely to be diverted for cell growth. It
bicarbonate buffer system to the left, eventually eliminating
is likely to be used for the synthesis of EPO instead. Flick-
3 from the medium. However, HCO3 is necessary for
inger et al. [40] and Leno et al. [41] also suggested that addi-
the good growth in CHO cells, because H+ transport system
tional ATP could accelerate energy-consuming steps in bio-
of CHO cells requires Na+, Cl−, and HCO −
3 [42]. Therefore,
synthetic pathway of protein.
the absence of atmospheric CO2 inhibits cell growth. Never-
theless, it must be worth considering a free-CO2 condition
Effect of 40 mM Sodium Lactate Addition on r-CHO
within the limits of the possibility, since the use of atmos-
Cells in the Absence of Carbon Dioxide
pheric CO2 increases costs. In this study, we examined
whether the addition of 40 mM sodium lactate could im-
The pH of cell culture medium is controlled by CO2/ bi-
prove the growth of r-CHO cells in the absence of atmos-
carbonate buffer system (H2O + CO2 ↔ H2CO3 ↔ H+ +
pheric CO2. The ratio of viable cells in the absence of at-
3 ). The net result of increasing atmospheric CO2 is to
mospheric CO2 to in the presence of atmospheric CO2 is
depress the pH, so the effect of elevated CO2 tension is neu-
shown in Fig. 4A. In the control experiment, where no so-
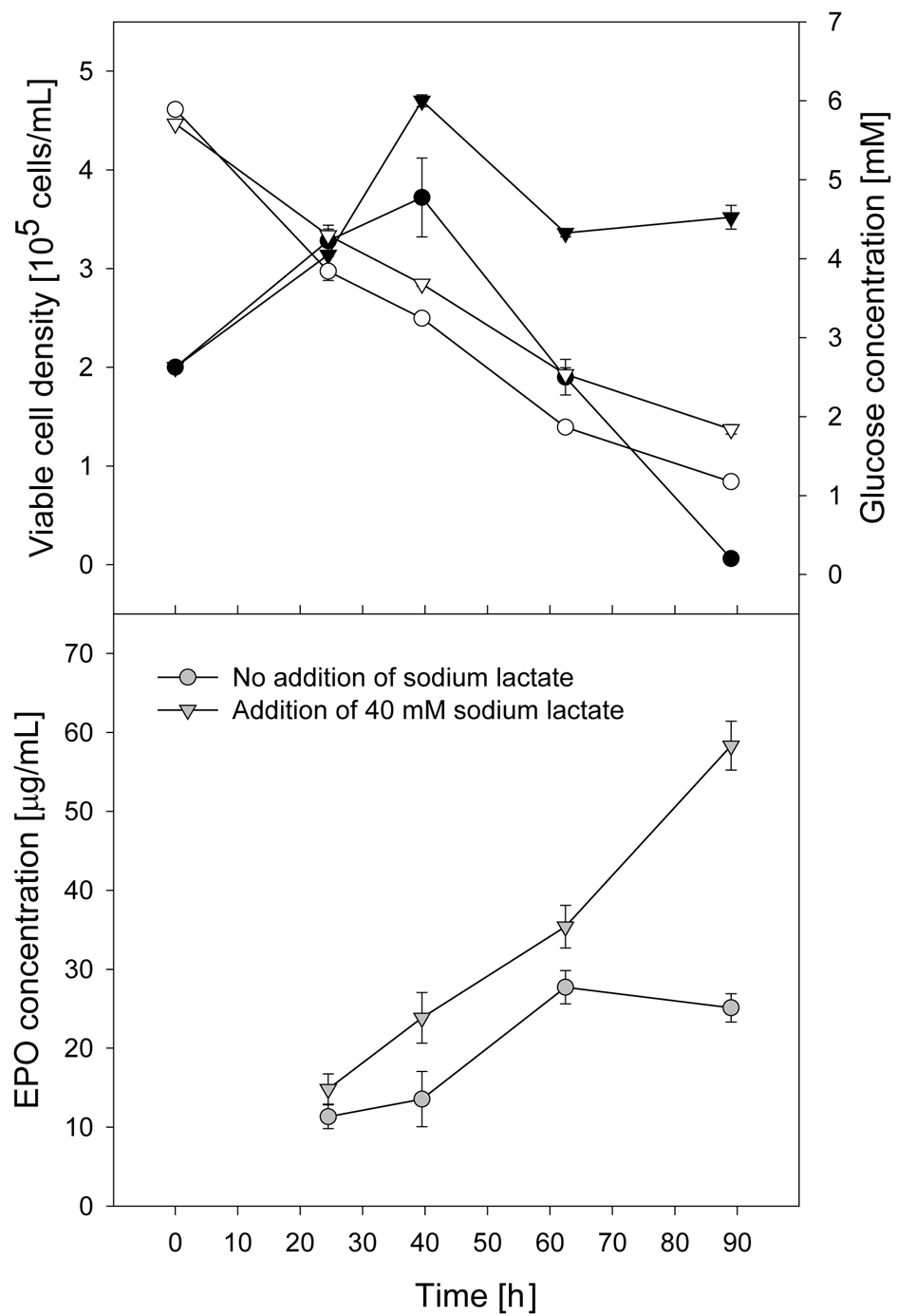
concentration in the presence of 40 mM sodium lactate was
232% of that in the absence of sodium lactate (Fig. 5B).
These results imply the positive effect of added 40 mM so-
dium lactate on the growth and EPO production of r-CHO
cells on the condition without atmospheric CO2.
Comparison between Sodium Chloride and Sodium
Lactate Addition in r-CHO Cell Cultures
The addition of sodium lactate increases the osmolarity,
for example, at the addition of 40 mM sodium lactate, the
osmolarity is approximately 400 mOsm/kg. However, the
osmolarity in the range of 270 330 mOsm/kg is known to be
quite acceptable for most cells [43]. In addition, growth de-
pression in hypertonic medium has been reported for MCL1
cells and other hybridoma cells [44,45]. Therefore, we had to
investigate whether the results in Figs. 2 and 3 were due to
the sodium lactate addition itself or the osmolarity induced
by the addition of sodium lactate. In advance of this investi-
gation, we examined the effect of medium osmolarity on cell
growth and EPO production of r-CHO cells over the range of
325 to 500 mOsm/kg. With an increase of the medium
osmolarity, the specific growth rate and maximum cell
density decreased (Fig. 6A). The specific growth rates at 380,
420, and 450 mOsm/kg decreased by 16, 31, and 46% as
compared with the control (325 mOsm/kg), respectively.
Fig. 5. Viable cell density (A, closed symbols), glucose concen-
The inhibition of cell growth was extreme at 500 mOsm/kg.
tration (A, open symbols), and EPO production (B) of r-
The result at 500 mOsm/kg could partially explain the
CHO cells in MEM-α supplemented with 0 (circle symbol)
exceptional phenomena observed in Figs. 2B and 3B when
or 40 (reversed triangle symbol) mM sodium lactate for 4
80 mM sodium lactate (approximately 480 mOsm/kg) was
days. Cells were inoculated at 2 × 105 cells/mL in 6-well
added. The result of cell growth in Fig. 6A was somewhat
plates and cultured in the absence of atmospheric CO2.
different from the observation by Kurano et al. [46] that
CHO cell growth was not affected by osmolarity below 390
mOsm/kg. In general, hybridomas are known to exhibit an
dium lactate was added, the viable cell density in the absence
increased specific antibody production rate when subjected
of atmospheric CO2 decreased by 51.9% compared with that
to environmental stress, as hyperosmotic pressure [26,44,
in the presence of atmospheric CO2 at the culture time of
45,47-56]. However, the increase of osmolarity decreases the
39.5 h and decreased significantly by 74.9% at 62.5 h. How-
specific growth rate [44,57,58]. Therefore, some "trade-off"
ever, the viable cell density in the absence of atmospheric
relationship between cell growth and protein production is
CO2 was 88.7% of that in the presence of atmospheric CO2
likely to exist [59]. We observed a similar response for the
at 39.5 h on the condition that 40 mM of sodium lactate was
specific EPO production of r-CHO cells (Fig. 6B). However,
present. The addition of 40 mM sodium lactate also caused
Glacken et al. observed a decrease of specific antibody pro-
the small change of external pH by reducing lactate produc-
duction rate in CRL-1606 cells under osmolarity conditions
tion, as shown in Fig. 4B. In other words, the added sodium
increased by the addition of sodium lactate [35]. The in-
lactate is likely to replace the role of atmospheric CO2 by
fluence of osmolarity on protein productivity seems to be
decreasing lactate accumulation. When atmospheric CO2
cell line dependent [60]. Increasing osmolarities from 325 to
was absence, the poor viability in the medium without so-
420 mOsm/kg increased the specific EPO production rate, as
dium lactate decreased dramatically to zero, as shown in Fig.
shown in Fig. 6B. The specific EPO production rate in cells
5A. This result indicates that the absence of CO2 inhibits the
exposed to 420 mOsm/kg hyperosmotic stress was increased
growth of r-CHO cells. However, r-CHO cells in the culture
by 74% when compared with that of cells in the culture with
medium containing 40 mM sodium lactate remained at the
325 mOsm/kg, and the specific EPO production rates be-
concentration of 3.52 × 105 cells/mL even at the culture time
tween 420 and 470 mOsm/kg formed a plateau. However, at
of 89 h. In addition, the glucose consumption in the presence
500 mOsm/kg, the specific EPO production rate dropped to
of 40 mM sodium lactate was slower than that in the absence
85.8% of 420 mOsm/kg. Oh et al. demonstrated that the
of sodium lactate. This result indirectly shows that the effi-
osmolarity effect acted quite specifically on Na+-dependent
ciency of energy production is increased by the addition of
transport systems, while there was no significant increase in
sodium lactate even on the condition without atmospheric
the uptake by Na+-independent transport systems [50]. Ac-
CO2. Moreover, because of the elongated culture span, EPO cordingly, the increase in osmolarity might enhance the
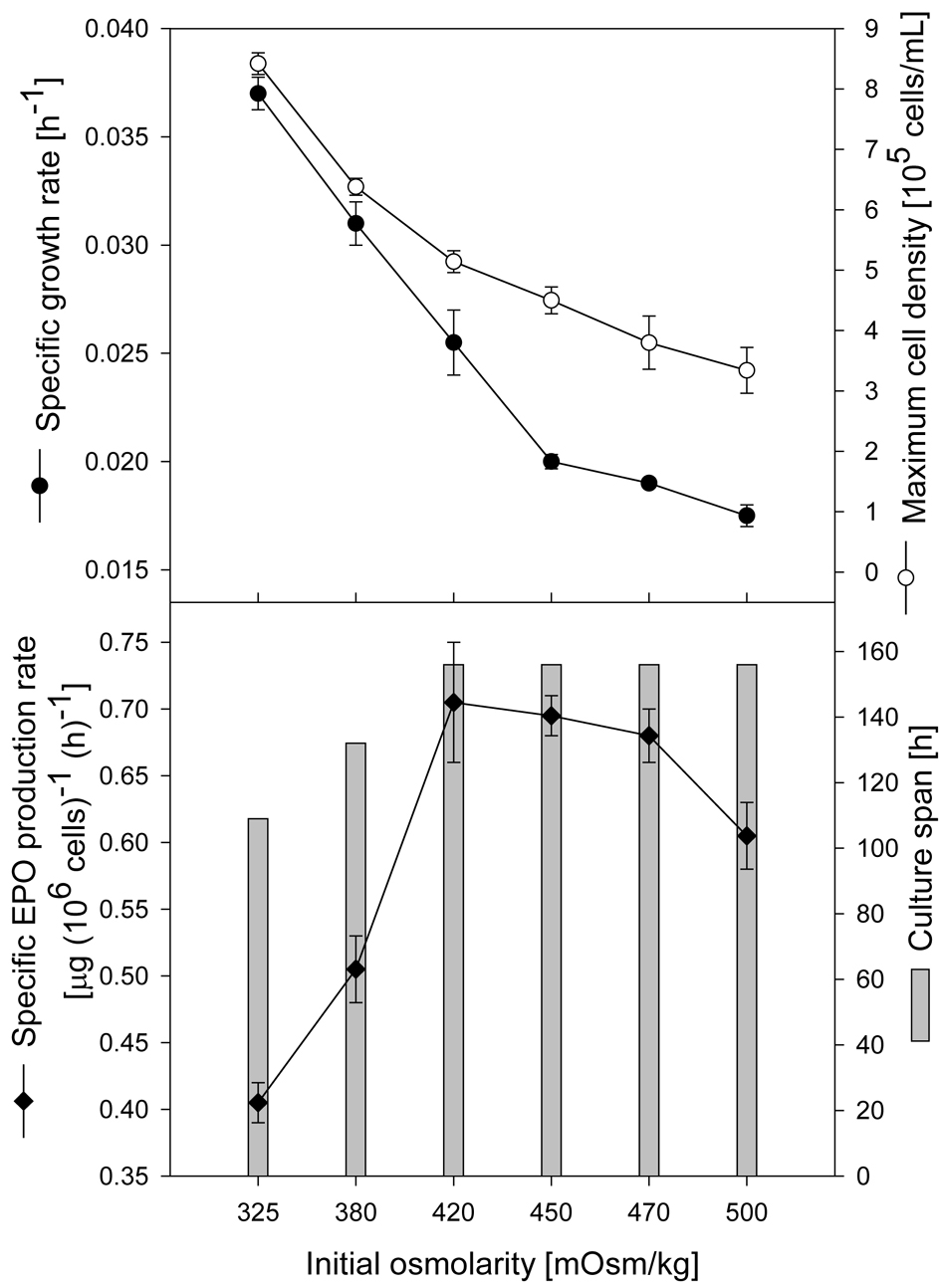
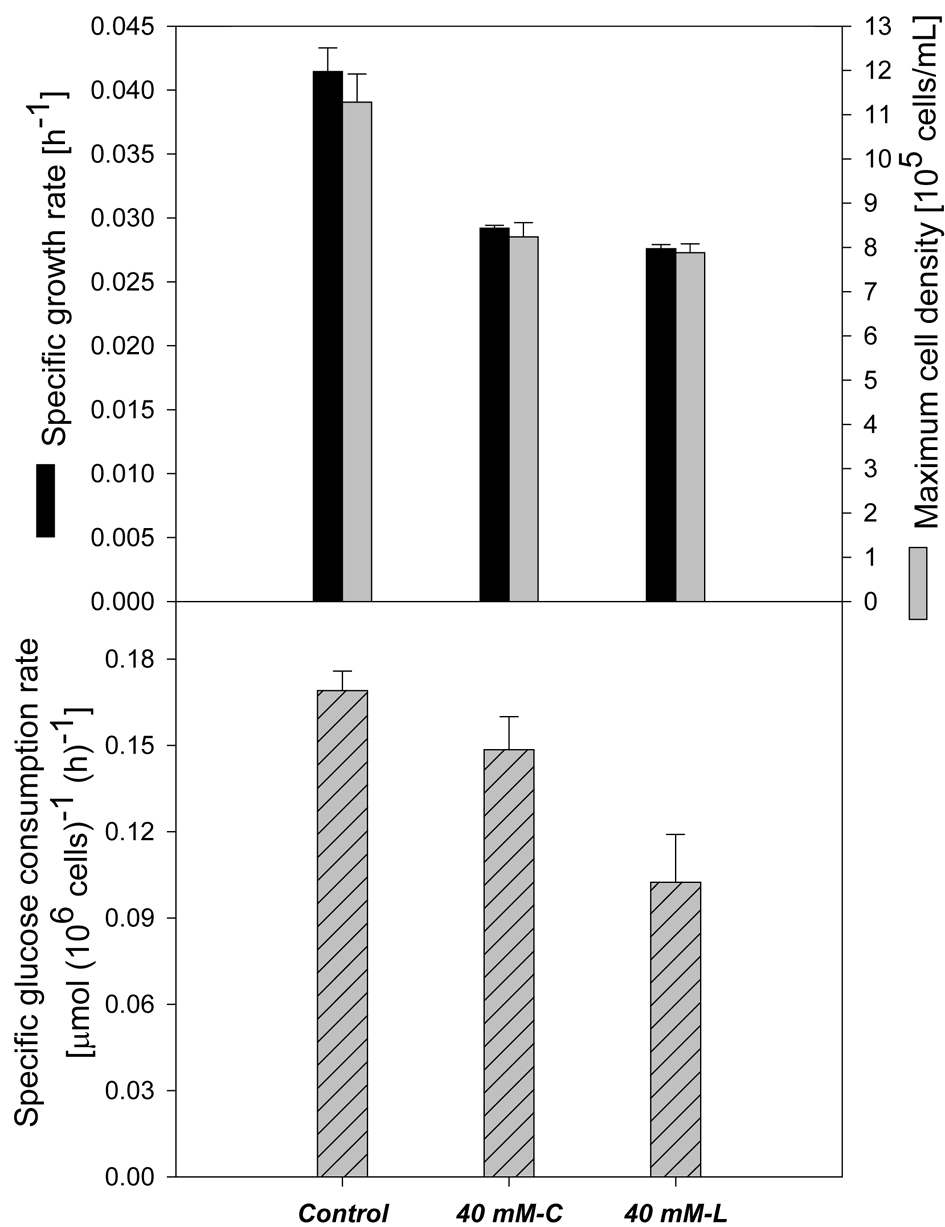
Biotechnol. Bioprocess Eng. 67=
Fig. 6. Effect of medium osmolarity on cell growth (A) and EPO
Fig. 7. Comparison of cell growth (A) and specific glucose con-
production (B) of r-CHO cells in MEM-α. Cells were in-
sumption rate (B) of r-CHO cells in MEM-α supplemented
oculated at 1 × 105 cells/mL and grown in 6-well plates
with 40 mM sodium chloride (40 mM-C) or 40 mM so-
for 7 days. The growth medium became hyperosmotic
dium lactate (40 mM-L) for 7 days. No supplement includ-
through the addition of sodium chloride.
ing sodium chloride and sodium lactate was added in the
control experiment. Cells were inoculated at 1 × 105
cells/mL in 6-well plates.
transport of nutrients, in particular, amino acids through Na+-
dependent transport systems [49]. In addition, stressed cul-
tures contain the enhanced levels of total RNA, of which
ity by comparing the results under the same osmolarity. In
80% is ribosomal RNA (rRNA). Higher rRNA content
result, we obtained no significant difference of cell growth
could increase the translation rate of protein. Oh et al. also
between lactate-containing culture and lactate-excluding
reported that RNA/DNA ratio was always higher for cultures
culture of the same osmolarity, as illustrated in Fig. 7A.
with higher osmolarity. High RNA/DNA ratio gives us a
Thus, it is obvious that lactate itself has very little influence
crude indication that transcription levels might be elevated in
on growth and the inhibition to cell growth is mainly due to
osmotically stressed cells [50]. Furthermore, under the
osmolarity. However, Fig. 7B showed that the specific glu-
higher osmolarity, histones are more readily dissociated
cose consumption rate decreased by 39.4% in addition of 40
from DNA, thus allowing easier accessibility of the chroma-
mM sodium lactate and 12.1% in the same concentration of
tin structure to RNA polymerase for transcription [61]. It
sodium chloride. In addition, specific lactate production rate
was also reported that the rate of transcription increased
was also found to be inhibited by 57.7% in 40 mM sodium
through the nucleosome at high osmolarity [62]. These re-
lactate and 24.7% in 40 mM sodium chloride (Fig. 8B).
ports can partially explain the reason why the increase in
Namely, when 40 mM sodium lactate was added, the spe-
osmolarity enhances the specific EPO production rate in our
cific glucose consumption rate and lactate production rate
were considerably lower than that observed in the equivalent
To verify that the increase of specific EPO productivity
concentration of sodium chloride. This indicates that the
observed in Fig. 2B was the effect of sodium lactate rather
effects of lactate on specific glucose consumption rate and
than the osmolarity effect, 40 mM sodium lactate and so-
lactate production rate are not due to the osmolarity induced
dium chloride were used for adjusting medium osmolarity to
by lactate addition alone. On any osmolarity adjusted by
approximately 400 mOsm/kg. We estimated that the effects
sodium chloride, YL/G remained constant in the range of
of lactate observed in this study were mainly related to the
1.8 2.0 (data not shown). This result agrees with that from
alteration in cell metabolism by sodium lactate itself rather
the report of Ryu and Lee [63]. However, on the condition
than the change in medium osmolarity induced by the added
that sodium lactate was added, YL/G decreased as concentra-
sodium lactate. Therefore, we attempted to separate the
tion of sodium lactated increased, as shown in Fig. 3C. It is
chemical effect of sodium lactate and the effect of osmolar-
assumed that osmolarity drives the effect of lactate on cell
effects of sodium lactate itself and osmolarity induced by it.
Therefore, the effects of sodium lactate may be partly ex-
plained by the increased osmolarity in the case of r-CHO
cells. After "correcting" for osmolarity effects, sodium lac-
tate itself was found to increase the specific EPO productiv-
ity by 98%, and to decrease the specific glucose consump-
tion rate and specific lactate production rate by 27 and 33%,
respectively. If osmolarity was the only effect, the results of
sodium lactate and sodium chloride observed in Figs. 7 and 8
would always be equal, but those of sodium lactate and so-
dium chloride did not coincide.
Relationship between Lactate Dehydrogenase
Activity and Lactate Addition in r-CHO Cell Culture
Lactate dehydrogenase catalyzes the interconversion of
lactate and pyruvate. The enzyme is a tetrameric protein [64]
and gives rise to five isoenzymes. In massive documentation
that has followed the reports of Dewey and Conklin [65] and
Van der Helm [66], it is generally agreed that human tissue
can be grouped into three categories based on LDH isoen-
zymes contents. LDH-1, -2, and -3 are prominent in tissues
such as heart, kidney, brain, pancreas, erythrocytes, lympho-
Fig. 8. Comparison of EPO (A) and lactate production (B) of r-
cytes, platelets, and diaphragm. LDH-4 and LDH-5 are the
CHO cells in MEM-α supplemented with 40 mM sodium
major fractions of liver, skeletal muscle, and granulocytes.
chloride (40 mM-C) or 40 mM sodium lactate (40 mM-L)
Prostate, thyroid, lung, adrenal, gastric mucosa, and ovary
for 7 days. No supplement including sodium chloride and
are characterized by preponderance of fractions intermediate
sodium lactate was added in the control experiment.
to the other groups [67]. H represents the peptide associated
Cells were inoculated at 1 × 105 cells/mL in 6-well plates.
with the main cardiac enzyme (LDH-1) and M the peptide
from skeletal muscle (LDH-5). The intermediate isoenzymes
LDH-2, -3, and -4 are hybrids formed by random association
growth, but lactate itself is the main reason for the specific of H and M subunits into tetramers. Thus, LDH-2, -3, and -4
glucose consumption rate and lactate production rate. In ad-
would contain H3M, H2M2, and HM3 subunits [68]. LDH-1
dition, the change of pH in culture medium during the expo-
moves farthest toward the anode during electrophoresis at
nential phase was 0.16 from initial pH in 40 mM sodium pH 8.6 and LDH-5 is the extreme cathodic enzymes. LDH-1
lactate and 0.25 in the same concentration of sodium chlo-
and LDH-2 are sometimes termed the fast isoenzymes and
ride (Fig. 8B). This indicates that sodium lactate addition can LDH-4 and LDH-5 the slow enzymes. LDH-3 is an enzyme
prevent the big drop of medium pH. The pH stabilization with intermediate electrophoretic mobility. LDH-X migrates
induced by sodium lactate may be profitable for the long-
between LDH-3 and LDH-4 on agarose gel [69]. Agarose
term cultures of r-CHO cells. We also observed that the gel electrophoresis of LDH isoenzyme pattern in r-CHO
addition of sodium lactate had the highest level of specific cells is shown in Fig. 9. We observed no change of LDH
EPO production rate among all the experiments tested, as isoenzyme pattern between lactate-containing culture and
shown in Fig. 8A. This increase in EPO productivity, on the lactate-excluding culture of r-CHO cells. Thus, it is obvious
condition that 40 mM sodium lactate was added, was not that lactate itself has no influence on the isoenzyme pattern
identical to the corresponding increase caused by the of LDH. Electrophoretic band for LDH isoenzyme type of r-
osmolarity. Specific EPO production rate increased by 144% CHO cells was present in the LDH-3 and LDH-4 regions,
in 40 mM sodium lactate and 46% in the same concentration whereas Blanco and Zinkham [69] observed that human ma-
of sodium chloride as compared with the control. In addition, ture ovary tissue had five isoenzymes with the exception of
the final accumulated EPO concentration from 40 mM so-
extra isoenzyme, LDH-X. Taken together, our result ob-
dium chloride supplemented culture showed the slightly tained in r-CHO cells originated from Chinese hamster ovary
higher level than that of the control. However, the accumu-
tissue is quite different from that of human mature ovary
lated EPO in 40 mM sodium lactate was 1.9 times higher than tissue. We conclude from Fig. 9 that LDH isoenzyme type of
that in the control. This proves indirectly that the increased r-CHO cells is LDH-X. It is generally known that H-type
osmolarity of culture medium is not the only effector on LDH is better adapted to function in the oxidation of lactate
EPO production. Although the exact mechanisms of actions to pyruvate, whereas M-type LDH is more suited to catalyze
by the two-stress factors, sodium lactate itself and osmolarity, the reverse reaction [70]. However, Brooks et al. [71] have
are unknown, it is evident that the influences of lactate addi-
reported that lactate oxidation exceeded pyruvate reduction
tion on EPO production and metabolisms are the combined by 10 40% in mitochondria isolated from liver, skeletal
Biotechnol. Bioprocess Eng. 69=
Fig. 9. Agarose gel electrophoretic pattern of LDH of r-CHO
cells in MEM-α supplemented with 40 mM sodium chlo-
ride (lanes 3 and 6) or 40 mM sodium lactate (lanes 4
and 7). No supplement including sodium chloride and
sodium lactate was added in the isoenzyme-determina-
tion of control (lanes 2 and 5). Cells were cultured for 27
(lanes 2, 3, and 4) and 92 (lanes 5, 6, and 7) h, respec-
tively. Cells were inoculated at 1 × 105 cells/mL in 100
mm dishes. Lactate dehydrogenase isoenzymes were
separated by electrophoresis on 1% agarose gel. A
marker (lanes 1 and 8) containing 5 human lactate dehy-
drogenase isoenzymes was used to aid in the identifica-
tion of sample LDH isoenzymes separated by electropho-
muscle, and heart. Heart and muscle mitochondria were
noted by the prevalence of both LDH-1 (H4) and LDH-5
(M4), while liver mitochondria were distinguished by the
Fig. 10. Lactate dehydrogenase (LDH) activity based on the
presence of LDH-5 (M4). Taking these reports together, it is
oxidation of lactate to pyruvate (A) and the reduction of
likely that the ability to convert lactate to pyruvate is not
pyruvate to lactate (B) in r-CHO cells. LDH activity was
necessarily governed by only LDH isoenzyme pattern.
measured after 27 and 92 h. Cells were inoculated at 1
Therefore, we expected that r-CHO cells would not only
× 105 cel s/mL in 100 mm dishes and cultured in MEM-α
reduce pyruvate but also oxidize lactate well despite LDH
supplemented with 40 mM sodium chloride or 40 mM
isoenzyme type of r-CHO cells presented between the LDH-
sodium lactate. No supplement including sodium chlo-
3 and LDH-4 types.
ride and sodium lactate was added in the control ex-
Figs. 10A or 10B shows LDH activities based on the oxi-
dation of lactate to pyruvate or the reduction of pyruvate to
lactate, respectively. LDH activities in Fig. 10 were obtained
to a similar pattern regardless of contrary reactions. It is
result in sodium chloride addition was preferably close to the
shown in Figs. 10A and 10B that LDH activity of r-CHO
control. The results above prove indirectly that the results
cells added 40 mM sodium lactate is higher than that in both
obtained by addition of sodium lactate differ from others in
the control and the 40 mM sodium chloride addition. How-
many respects because of lactate effect in itself rather than
ever, the difference of LDH activities at 27 h-culture time is
only osmolarity effect. The measured activity in vitro of
much smaller than that at 92 h-culture time. One of differ-
LDH enzyme, which was obtained by the addition of 40 mM
ences between 27 and 92 h-culture time is the remained con-
sodium lactate, was high in the assay based on the reduction
centration of glucose that is a major carbon source in culture
of pyruvate (Fig. 10B) as well as in the assay based on the
medium. About 4 mM of glucose remained at 27 h-culture
oxidation of lactate (Fig. 10A). Then, why did lactate pro-
time, while glucose was completely exhausted at 92 h-
duction decrease in the culture with sodium lactate? The
culture time (data not shown). This indicates that the notice-
reason might be attributed to the difference between in vitro
able enhancement of LDH activity by the added lactate does
LDH assay condition and in vivo cell culture condition. The
not occur in the presence of glucose, but the added lactate
concentration of pyruvate used in LDH assay, based on the
can influence LDH activity greatly when glucose is almost
reduction of pyruvate, was 22.7 mM and there was no addi-
consumed. The addition of 40 mM sodium chloride, which
tion of lactate. However, the used culture medium contained
had same osmolarity as 40 mM sodium lactate, did not en-
1 mM pyruvate and 40 mM sodium lactate. In other words,
hance LDH activity like the addition of lactate. The when lactate concentration was low (nearly zero), LDH in r-
CHO cells would mainly catalyze the reduction of pyruvate.
ovary cells cultured in serum-free media. Biotechnol.
However, on the condition that high concentration (40 mM
Bioprocess Eng. 11: 319-324.
in this study) of lactate was added, LDH was likely to cata-
2. Kim, J. S., M. K. Min, and E. C. Jo (2001) High-level
lyze the oxidation of lactate at a considerable rate, since en-
expression and characterization of single chain urokinase-
zyme activity could be varied despite the same enzyme when
type plasminogen activator (scu-PA) produced in recom-
the environment of enzyme reaction changed. Therefore, a
binant Chinese hamster ovary (CHO) cells. Biotechnol.
considerable amount of lactate accumulation (about 10 mM)
Bioprocess Eng. 6: 117-127.
was in the control experiment contained only 1 mM pyruvate
3. Kato, H., T. Inoue, N. Ishii, Y. Murakami, M. Matsu-
without the addition of lactate, and only 5 mM of lactate
mura, T. Seya, and P. C. Wang (2002) A novel simple
accumulation was observed in the case of the addition of 40
method to purify recombinant soluble human comple-
mM sodium lactate (data not shown). From the results above,
ment receptor type 1 (sCR1) from CHO cell culture.
we also explained why specific glucose uptake rate de-
Biotechnol. Bioprocess Eng. 7: 67-75.
creased by the addition of 40 mM sodium lactate. LDH of r-
4. Chang, K. H., K. S. Kim, and J. H. Kim (1998) Analy-
CHO cells is activated by the addition of 40 mM sodium
sis of erythropoietin glycoform produced by recombi-
lactate in our study. This activated LDH catalyzes the oxida-
nant CHO cells using the lectin-blotting technique.
tion of lactate to pyruvate with simultaneous reduction of
Biotechnol. Bioprocess Eng. 3: 40-43.
NAD to NADH, which will lead to a higher accumulation of
5. Bae, G. W., D. W. Jeong, H. J. Kim, G. M. Lee, H. W.
NADH than in the control. The somewhat increased concen-
Park, T. B. Choe, S. M. Kang, I. Y. Kim, and I. H. Kim
tration of NADH will lower the specific consumption rate of
(2006) High productivity of t-PA in CHO cells using
glucose in our study, since glycolysis is inhibited at the pres-
hypoxia response element. J. Microbiol. Biotechnol.
ence of excess NADH.
6. Li, J., C. Menzel, D. Meier, C. Zhang, S. Dubel, and T.
Jostock (2007) A comparative study of different vector
designs for the mammalian expression of recombinant
IgG antibodies. J. Immunol. Methods 318: 113-124.
We suggest that the main reason for results obtained in
7. Choi, Y. S., D. Y. Lee, I. Y. Kim, S. Kang, K. Ahn, H. J.
our study is a series of alterations of metabolism by the lac-
Kim, Y. H. Jeong, G. T. Chun, J. K. Park, and I. H. Kim
tate addition. Sodium lactate added with 40 mM lets a LDH
(2000) Ammonia removal using hepatoma cells in
enzyme catalyze the oxidation of lactate to pyruvate at a
mammalian cell cultures. Biotechnol. Prog. 16: 760-768.
relatively high rate, so the accumulation of NADH is in-
8. Kim, N. Y., Y. J. Lee, H. J. Kim, J. H. Choi, J. K. Kim,
creased. The uptake rate of glucose becomes slow because of
K. H. Chang, J. H. Kim, and H. J. Kim (2004) En-
the NADH accumulation. The decreased consumption rate
hancement of erythropoietin production from Chinese
of glucose causes a high-energy yield and a small amount of
hamster ovary (CHO) cells by introduction of the urea
lactate accumulation, and then the acidification of a culture
cycle enzymes, carbamoyl phosphate synthetase 1 and
medium is suppressed. In addition, the pyruvate produced by
ornithine transcarbamylase. J. Microbiol. Biotechnol.
the oxidation of lactate is used for production of energy and
formation of various amino acids. Consequently, EPO pro-
9. Lao, M. S. and D. Toth (1997) Effects of ammonium
duction is enhanced at 40 mM lactate addition, since r-CHO
and lactate on growth and metabolism of a recombinant
cells can synthesize more proteins through enough amino
Chinese hamster ovary cell culture. Biotechnol. Prog.
acids and energy. By using this approach, the cells can be
maintained viable for a longer period with good growth. In
10. Omasa, T., K. Higashiyama, S. Shioya, and K. Suga
result, a higher product concentration can be anticipated.
(1992) Effects of lactate concentration on hybridoma
culture in lactate-controlled fed-batch operation. Bio-
technol. Bioeng. 39: 556-564.
Acknowledgements This work was supported by grants
11. Ozturk, S. S., M. R. Riley, and B. O. Palsson (1992)
from the Basic Research Program of the Korea Science and
Effects of ammonia and lactate on hybridoma growth,
Engineering Foundation (97-0502-0101-3), the Ministry of
metabolism, and antibody production. Biotechnol. Bio-
Commerce, Industry and Energy (10006753-2006-22), and
eng. 39: 418-431.
the Rural Development Administration (20050301034477).
12. Hassell, T., S. Gleave, and M. Butler (1991) Growth
inhibition in animal cell culture. The effect of lactate and
ammonia. Appl. Biochem. Biotechnol. 30: 29-41.
Received November 28, 2006; accepted February 1, 2007
13. Kurano, N., C. Leist, F. Messi, S. Kurano, and A.
Fiechter (1990) Growth behavior of Chinese hamster
ovary cells in a compact loop bioreactor. 2. Effects of
medium components and waste products. J. Biotechnol.
1. Park, H., S. An, and T. Choe (2006) Change of insulin-
14. Glacken, M. W., E. Adema, and A. J. Sinskey (1988)
like growth factor gene expression in Chinese hamster
Mathematical descriptions of hybridoma culture kinet-
Biotechnol. Bioprocess Eng. 71=
ics: I. Initial metabolic rates. Biotechnol. Bioeng. 32:
stimulating factor or interleukin-3, and a subline requir-
ing erythropoietin. Blood 78: 2261-2268.
15. Reuveny, S., D. Velez, J. D. Macmillan, and L. Miller
29. Mosmann, T. (1983) Rapid colorimetric assay for cellu-
(1987) Factors affecting monoclonal antibody produc-
lar growth and survival: Application to proliferation and
tion in culture. Dev. Biol. Stand. 66: 169-175.
cytotoxicity assays. J. Immunol. Methods 65: 55-63.
16. Miller, W. M., C. R. Wilke, and H. W. Blanch (1988)
30. Donnelly, M. and I. E. Scheffler (1976) Energy metabo-
Transient responses of hybridoma cells to lactate and
lism in respiration-deficient and wild type Chinese ham-
ammonia pulse and step changes in continuous culture.
ster fibroblasts in culture. J. Cell Physiol. 89: 39-51.
Bioprocess Eng. 3: 113-122.
31. Kimura, R. and W. M. Miller (1996) Effects of elevated
17. Singh, R. P., M. Al-Rubeai, C. D. Gregory, and A. N.
pCO2 and/or osmolality on the growth and recombinant
Emery (1994) Cell death by necrosis and apoptosis dur-
tPA production of CHO cells. Biotechnol. Bioeng. 52:
ing the culture of commercially important cell lines. pp
187-191. In: R. E. Spier, J. B. Griffiths, and W. Berthold
32. Madshus, I. H. (1988) Regulation of intracellular pH in
(eds.). Animal Cell Technology: Products of Today,
eukaryotic cells. Biochem. J. 250: 1-8.
Prospects for Tomorrow. Butterworth-Heinemann, Ox-
33. Gramer, M. J. and C. F. Goochee (1993) Glycosidase
activities in Chinese hamster ovary cell lysate and cell
18. Yang, M. and M. Butler (2000) Effects of ammonia on
culture supernatant. Biotechnol. Prog. 9: 366-373.
CHO cell growth, erythropoietin production, and gly-
34. Cruz, H. J., C. M. Freitas, P. M. Alves, J. L. Moreira,
cosylation. Biotechnol. Bioeng. 68: 370-380.
and M. J. T. Carrondo (2000) Effects of ammonia and
19. Porter, D. L. and M. A. Goldberg (1994) Physiology of
lactate on growth, metabolism, and productivity of BHK
erythropoietin production. Semin. Hematol. 31: 112-121.
cells. Enzyme Microb. Technol. 27: 43-52.
20. Miller, M. E., M, Rorth, H. H. Parving, D. Howard, I.
35. Glacken, M. W. (1988) Catabolic control of mammalian
Reddington, C. R. Valeri, and F. Stohlman, Jr. (1973)
cell culture. Bio/Technol. 6: 1041-1050.
pH effect on erythropoietin response to hypoxia. N. Engl.
36. Lanks, K. W. and P. W. Li (1988) End products of glu-
J. Med. 288: 706-710.
cose and glutamine metabolism by cultured cell lines. J.
21. Thorens, B. and P. Vassalli (1986) Chloroquine and
Cell Physiol. 135: 151-155.
ammonium chloride prevent terminal glycosylation of
37. Petch, D. and M. Butler (1994) Profile of energy me-
immunoglobulins in plasma cells without affecting se-
tabolism in a murine hybridoma: glucose and glutamine
cretion. Nature 321: 618-620.
utilization. J. Cell Physiol. 161: 71-76.
22. Glacken, M. W., R. J. Fleischaker, and A. J. Sinskey
38. Irani, N., M. Wirth, J. van Den Heuvel, and R. Wagner
(1986) Reduction of waste product excretion via nutrient
(1999) Improvement of the primary metabolism of cell
control: Possible strategies for maximizing product and
cultures by introducing a new cytoplasmic pyruvate car-
cell yields on serum in cultures of mammalian cells.
boxylase reaction. Biotechnol. Bioeng. 66: 238-246.
Biotechnol. Bioeng. 28: 1376-1389.
39. Ljunggren, J. and L. Haggstrom (1994) Catabolic con-
23. Reitzer, L. J., B. M. Wice, and D. Kennell (1979) Evi-
trol of hybridoma cells by glucose and glutamine limited
dence that glutamine, not sugar, is the major energy
fed batch cultures. Biotechnol. Bioeng. 44: 808-818.
source for cultured HeLa cells. J. Biol. Chem. 254:
40. Flickinger, M. C., N. K. Goebel, T. Bibila, and S.
Boyce-Jacino (1992) Evidence for posttranscriptional
24. Eagle, H., S. Barban, M. Levy, and H. O. Schulze
stimulation of monoclonal antibody secretion by L-
(1958) The utilization of carbohydrates by human cell
glutamine during slow hybridoma growth. J. Biotechnol.
cultures. J. Biol. Chem. 233: 551-558.
25. Xie, L. and D. I. C. Wang (1994) Fed-batch cultivation
41. Leno, M., O. W. Merten, and J. Hache (1992) Kinetic
of animal cells using different medium design concepts
analysis of hybridoma growth and monoclonal antibody
and feeding strategies. Biotechnol. Bioeng. 95: 270-284.
production in semicontinuous culture. Biotechnol. Bio-
26. Miller, W. M., H. W. Blanch, and C. R. Wilke (1988) A
eng. 39: 596-606.
kinetic analysis of hybridoma growth and metabolism in
42. Reusch, H. P., J. Lowe, and H. E. Ives (1995) Osmotic
batch and continuous culture: effect of nutrient concen-
activation of a Na+-dependent Cl−/HCO −
3 exchanger. Am.
tration, dilution rate, and pH. Biotechnol. Bioeng. 32:
J. Physiol. 268: C147-C153.
43. Freshney, R. I. (1994) Culture of Animal Cells: A Man-
27. Ozturk, S. S. and B. O. Palsson (1991) Growth, meta-
ual of Basic Technique. Wiley-Liss, New York, NY,
bolic, and antibody production kinetics of hybridoma
cell culture: 1. Analysis of data from controlled batch
44. Oyaas, K., T. M. Berg, O. Bakke, and D. W. Levine
reactors. Biotechnol. Prog. 7: 471-480.
(1989) Hybridoma growth and antibody production un-
28. Chiba, S., F. Takaku, T. Tange, K. Shibuya, C. Misawa,
der conditions of hyperosmotic stress. pp. 212-220. In:
K. Sasaki, K. Miyagawa, Y. Yazaki, and H. Hirai (1991)
R. E. Spier, J. B. Griffiths, and P. J. Crooy (eds.). Ad-
Establishment and erythroid differentiation of a cyto-
vances in Animal Cell Biology and Technology for Bio-
kine-dependent human leukemic cell line F-36E: A pa-
processes. Butterworth, Kent, UK.
rental line requiring granulocyte-macrophage colony-
45. Oyaas, K., T. E. Ellingsen, N. Dyrset, and D. W. Levine
(1994) Utilization of osmoprotective compounds by hy-
Prog. 19: 1734-1741.
bridoma cells exposed to hyperosmotic stress. Biotech-
58. Kim, N. S. and G. M. Lee (2002) Response of recom-
nol. Bioeng. 43: 77-89.
binant Chinese hamster ovary cells to hyperosmotic
46. Kurano, N., C. Leist, F. Messi, S. Kurano, and A.
pressure: effect of Bcl-2 overexpression. J. Biotechnol.
Fiechter (1990) Growth behavior of Chinese hamster
ovary cells in a compact loop bioreactor. 1. Effects of
59. Berg, T. M., K. Oyaas, and D. W. Levine (1990)
physical and chemical environments. J. Biotechnol. 15:
Growth and antibody production of hybridoma cells ex-
posed to hyperosmotic stress. pp. 93-97. In: H. Mura-
47. Bibila, T. A., C. S. Ranucci, K. Glazomitsky, B. C.
kami (ed.). Trends in Animal Cell Culture Technology.
Buckland, and J. G. Aunins (1994) Monoclonal anti-
VCH, New York, NY, USA.
body process development using medium concentrates.
60. Lee, G. M. and S. Y. Park (1995) Enhanced specific
Biotechnol. Prog. 10: 87-96.
antibody productivity of hybridomas resulting from hy-
48. Nuss, D. L. and G. Koch (1976) Variation in the relative
perosmotic stress is cell line-specific. Biotechnol. Lett.
synthesis of immunoglobulin G and non-immunoglo-
bulin G proteins in cultured MPC-11 cells with changes
61. Lilley, D. M., M. F. Jacobs, and M. Houghton (1979)
in the overall rate of polypeptide chain initiation and
The nature of the interaction of nucleosomes with a
elongation. J. Mol. Biol. 102: 601-612.
eukaryotic RNA polymerase II. Nucleic Acids Res. 7:
49. Oh, S. K. W., P. Vig, F. Chua, W. K. Teo, and M. G. S.
Yap (1993) Substantial overproduction of antibodies by
62. Walter, W. and V. M. Studitsky (2001) Facilitated
applying osmotic pressure and sodium butyrate. Bio-
transcription through the nucleosome at high ionic
technol. Bioeng. 42: 601-610.
strength occurs via a histone octamer transfer mecha-
50. Oh, S. K. W., F. K. F. Chua, and A. B. H. Choo (1995)
nism. J. Biol. Chem. 276: 29104-29110.
Intracellular responses of productive hybridomas sub-
63. Ryu, J. S. and G. M. Lee (1997) Effect of hypoosmotic
jected to high osmotic pressure. Biotechnol. Bioeng. 46:
stress on hybridoma cell growth and antibody produc-
tion. Biotechnol. Bioeng. 55: 565-570.
51. Oyaas, K., T. E. Ellingsen, N. Dyrset, and D. W. Levine
64. Huston, J. S., W. W. Fish, K. G. Mann, and C. Tanford
(1994) Hyperosmotic hybridoma cell cultures: Increased
(1972) Studies on the subunit molecular weight of beef
monoclonal antibody production with addition of gly-
heart lactate dehydrogenase. Biochemistry 11: 1609-
cine betaine. Biotechnol. Bioeng. 44: 991-998.
52. Ozturk, S. S. and B. O. Palsson (1991) Effect of medium
65. Dewey, M. M. and J. L. Conklin (1960) Starch gel elec-
osmolarity on hybridoma growth, metabolism, and anti-
trophoresis of lactic dehydrogenase from rat kidneys.
body production. Biotechnol. Bioeng. 37: 989-993.
Proc. Soc. Exp. Biol. Med. 105: 492-494.
53. Park, S. Y. and G. M. Lee (1995) Feasibility study on
66. Van der Helm, H. J. (1961) Simple method of demon-
the use of hyperosmolar medium for improved antibody
strating lactic acid dehydrogenase isoenzymes. Lancet 2:
production of hybridoma cells in a long-term, repeated-
fed batch culture. Bioprocess Eng. 13: 79-86.
67. Starkweather, W. H., H. H. Spencer, E. L. Schwarz, and
54. Reddy, S., K. D. Bauer, and W. M. Miller (1992) De-
H. K. Schoch (1966) The electrophoretic separation of
termination of antibody content in live versus dead hy-
lactate dehydrogenase isoenzymes and their evaluation
bridoma cells: Analysis of antibody production in os-
in clinical medicine. J. Lab. Clin. Med. 67: 329-343.
motically stressed cultures. Biotechnol. Bioeng. 40: 947-
68. Millar, D. B. S., M. R. Summers, and J. A. Niziolek
(1971) Spontaneous in vitro hybridization of LDH ho-
55. Reddy, S. and W. M. Miller (1994) Effects of abrupt and
mopolymers in the undenatured state. Nat. New Biol.
gradual osmotic stress on antibody production and con-
tent in hybridoma cells that differ in production kinetics.
69. Blanco, A. and W. H. Zinkham (1963) Lactate dehydro-
Biotechnol. Prog. 10: 165-173.
genase in human testes. Science 139: 601-602.
56. Sureshkumar, G. K. and R. Mutharasan (1991) The in-
70. Voet, D. and J. G. Voet (1995) Biochemistry. pp. 465.
fluence of temperature on a mouse-mouse hybridoma
John Wiley & Sons, New York, NY, USA.
growth and monoclonal antibody production. Biotechnol.
71. Brooks, G. A., H. Dubouchaud, M. Brown, J. P.
Bioeng. 37: 292-295.
Sicurello, and C. E. Butz (1999) Role of mitochondrial
57. Lee, M. S., K. W. Kim, Y. H. Kim, and G. M. Lee
lactate dehydrogenase and lactate oxidation in the intra-
(2003) Proteome analysis of antibody-expressing CHO
cellular lactate shuttle. Proc. Natl. Acad. Sci. USA 96:
cells in response to hyperosmotic pressure. Biotechnol.
Source: http://bbe.or.kr/storage/journal/BBE/12_1/6361/articlefile/article.pdf
Identification and management of Guillain-Barré syndrome in the context of Zika virus Interim guidance 25 February 2016 WHO/ZIKV/MOC/16.4 1. Introduction critical care physicians and nurses. This guidance may also be used by those responsible for developing local and 1.1 Background national health protocols and policies, and policy-makers in
1. Introduction 2. The Bacterial Balance within a chronic wound 2.1 Bacterial involvement in a chronic wound and assessment 2.2 Bacterial screening of a chronic wound 2.3 Variables that affect the bacterial burden of a wound 2.4 Bacterial burden, pathogenicity and the presence of biofilms in wound healing 2.5 Antibiotics and antiseptics in wound infection