Marcoinfussi.it
Plant Biology ISSN 1435-8603
Tetracycline accumulates in Iberis sempervirens L. throughapoplastic transport inducing oxidative stress and growthinhibitionG. Di Marco1, A. Gismondi1, L. Canuti1, M. Scimeca2, A. Volpe2 & A. Canini11 Department of Biology, University of Rome ‘Tor Vergata', Rome, Italy2 Department of Biomedicine and Prevention, University of Rome ‘Tor Vergata', Rome, Italy
Antibiotic bioaccumulation; apoplastictransport; confocal microscopy; environmental
Environmental antibiotic contamination is due mainly to improper and illegal
contamination; oxidative stress; tetracycline;
disposal of these molecules that, yet pharmacologically active, are excreted by humans
transmission electron microscopy.
and animals. These compounds contaminate soil, water and plants. Many studies havereported the bioaccumulation of antibiotics in plants and their negative effects on
photosynthesis, cell growth and oxidative balance. Therefore, the principal objective
A. Canini, Department of Biology, University
of this paper was the study of antibiotic accumulation sites in plants and its uptake
of Rome ‘Tor Vergata', Via della Ricerca
modality. Iberis sempervirens L., grown in soil and in agar in the presence or absence
Scientifica 1, 00133 Roma, Italy.
of tetracycline, were used as a model system. Using confocal and transmission electron
microscopy, we demonstrated that tetracycline was absorbed and propagated in plantsthrough apoplastic transport and also accumulated in intercellular spaces. Tetracy-
cline was rarely detected inside cells (in cytoplasm and mitochondria where, coherent
to its pharmacological activity, it probably affected ribosomes), except in stomata.
Moreover, we verified and clarified further the phytotoxic effects of tetracycline on
Received: 16 July 2013; Accepted: 23 July
plants. We observed that the antibiotic induced a large reduction in plant growth and
development and inhibition of photosynthetic activity. As tetracycline may lead tooxidative stress in plants, plant cells tried to balance this disequilibrium by increasing
the amount and activity of some endogenous enzyme antioxidant agents (superoxidedismutase 1 and catalase) and levels of antiradical secondary metabolites.
causing problems to aquatic organisms, animals, microorgan-
isms, humans and plants (Kumar et al. 2005b). In fact, antibi-
Over the last 50 years, public awareness of the long-term
otics can induce development of bacterial resistance (mutation
adverse effects of antibiotics on human health and ecology has
in genes of ‘resistance', transfer of resistance genes among
greatly increased, despite scientific evidence (Halling-Sørensen
microorganisms, increase in selection pressure for resistant
et al. 1998; Zuccato et al. 2006; Cox & Wright 2013; Xue et al.
organisms) and alterations in microbial communities, with
2013). Tetracycline (TC) is a broad-spectrum antibiotic largely
consequent alteration of nutrient cycles and decomposition of
employed in veterinary and human medicine for the treatment
organic matter (Jensen 2001). In humans, antibiotics can cause
and control of a wide variety of bacterial infections (Baguer
chronic or acute toxic effects or allergic reactions, e.g. intoler-
et al. 2000). Environmental contamination with pharmaceuti-
ance, urticaria, anaphylactic shock, bronchial asthma and
cal residues is determined in different ways, but essentially is
angioedema, because of overdose or low-level prolonged expo-
due to wastewater discharge and agricultural application of
sure (Sungpyo & Aga 2007). Finally, antibiotics can affect
sewage sludge and animal manure (Hirsch et al. 1999). In par-
plants through inhibition of photosynthesis, consistent
ticular, intensive animal farming implies considerable use of
decreases in growth, reduction in leaf length, reduction in root
antimicrobial drugs. These compounds are given to livestock as
number and elongation and an increase in levels of reactive
‘medicated' feed additives in order to prevent diseases (Kolpin
oxygen species (ROS) (Kapustka 1997). It is reported that
et al. 2002). Since only a small percentage of antibiotics are
plants grown on contaminated soils absorb antibiotics and
absorbed or degraded through metabolism, they are excreted
accumulate them in different organs, but the uptake modality,
with urine and faeces, as a mixture of bioactive molecules, in
the storage sites and the processing of these drugs in cells are
sewage systems and consequently in the environment (Carballa
not yet documented. Moreover, the major concern about anti-
et al. 2004; Glassmeyer et al. 2005). However, the introduction
biotic pollution in plants is relative contamination of the food
of such chemicals in the environment can be also associated
supply and consequent health risks (Migliore et al. 1996, 2010;
with animal waste application in field manuring because anti-
Kumar et al. 2005a; Dolliver et al. 2007). The aim of this work
biotics generally remain stable for a long time during storage
was to study the interaction between Iberis sempervirens L. and
(Halling-Sørensen et al. 1998; Jørgensen & Halling-Sørensen
TC. We chose this plant as a model system because it belongs
2000). These molecules contaminate the soil, plants and waters,
to Cruciferae, like Arabidopsis thaliana (L.) Heynh, the most
Plant Biology 2013 German Botanical Society and The Royal Botanical Society of the Netherlands
Tetracycline uptake and effects in plants
Di Marco, Gismondi, Canuti, Scimeca, Volpe & Canini
studied plant in plant biology, and because in the Botanical
size; Reprosil, Germany). Chromatographic separation was
Garden of Rome ‘Tor Vergata' we are also monitoring environ-
carried out with a mobile phase consisting of 0.1% formic acid
mental contamination, as in previous studies (Di Marco et al.
(v/v) (phase A) and acetonitrile (phase B) at a flow rate of
2012), through the detection of antibiotics in hive products of
0.3 mlmin 1. Elution started at 3% A solvent for 3 min and
honeybees (Apis mellifera ligustica Spinola) in foraging of this
then was linearly increased up to 95% over 16 min. Finally,
Mediterranean species. TC was chosen because it is the cheap-
the total run time, including cleaning and pre-equilibration
est and most easily available antibiotic for therapy in human
steps, was 30 min. Injection volume was 20 ll and column
and animal diseases, including bee treatment against Paeniba-
temperature was set at 30 °C. Mass spectrometry was carried
cillus larvae White (American foulbrood). In particular, we
out using a LCMS 2020 single quadrupole mass spectrometer
evaluated: (i) antibiotic absorption capacity from soil (detec-
(Shimadzu). The instrument was operated using electro-spray
tion and quantification); (ii) TC bioaccumulation sites; (iii)
ionisation (ESI) in positive ion mode. Data acquisition was
uptake mode (physiological and molecular); and (iv) plant
performed using LAB-SOLUTION software (Shimadzu). Ionisation
response to TC.
source parameters were: capillary voltage 3.0 kV, interfacevoltage 4.5 kV, heat block 200 °C, DL temperature 250 °C,nebulising gas 1.5 lmin 1 and drying gas flow 15 l 1 (N2). The
MATERIAL AND METHODS
TC standard was diluted in methanol and infused at flow rateof 0.3 mmin 1 in order to establish the pseudo-molecular ion
in a positive ESI mode and check for presence of potential
Iberis sempervirens was grown and treated with TC (Sigma-
impurity or adduct ions. Only protonated species [M+H]+
Aldrich, Milan, Italy) in the Botanical Garden of Rome ‘Tor
were observed for all the compounds. Data are expressed as lg
Vergata'. TC was dissolved in soil (100 and 200 mgl 1) and
of TC per kg of sample fresh weight (lgTCkg 1 FW).
adult plants were monitored for 30 days. Commercial seedswere sterilised with 5% sodium hypochlorite for 30 min. After
Confocal microscopy (CM)
many vigorous washings in sterile distilled water, seeds weretransferred into transparent plastic flasks (tissue culture flasks
Six plants for each treatment, grown on agar for 30 days, were
175 cm2, BD Falcon, Franklin Lakes, New Jersey, USA) con-
washed thoroughly with distilled water to remove soil from the
taining sterile agarised Murashige and Skoog culture medium
roots. Whole seedling was positioned on a microscope slide
(Sigma-Aldrich), with or without TC. TC was added to the
and observed, according to a modified method of Pautke et al.
medium at nominal concentrations of 100 and 200 mgl 1.
(2010), with a confocal laser scanning FV 1000 system with an
Plants were cultivated indoors under a natural photoperiod and
Olympus IX81 microscope (Olympus, Shinjuku-ku, Tokyo,
temperature (Rome, Italy, March–October). All procedures
Japan). TC (visible as yellow) was detected by capturing the
were performed in a biohazard cabinet and plants were main-
natural emission at 527 nm after excitation performed with a
tained in sealed flasks in sterile conditions throughout the
laser at 405 nm. For chlorophyll (detectable as red), the excita-
experiment. After 30 days, seedlings were harvested, weighed
tion was 635 nm and emission 668 nm. Iso-surface and 3-D
80 °C until chemical analysis. TC 100 and TC
volume digital images were captured at 209 enlargement and
200 are the code used in this work to identify TC treatments
analysed using IMARIS 7.4 (Bitplane, Zurich, Switzerland) soft-
with 100 and 200 mgl 1, respectively.
Tetracycline detection in plants
Transmission electron microscopy (TEM)
Tetracycline extraction was carried out according to Brambilla
Root portions and leaf samples were cut on and soaked in a
et al. (2007), with some modifications. A total of 2 g plant was
drop of 3% paraformaldehyde in 0.06 M Sørensen phosphate
finely homogenised and 5 ml citrate buffer, pH 4.7, added.
buffer (pH 7.2). Samples were then transferred into glass vials
Samples were cleaned-up with metal chelate affinity chroma-
and fixed for 90 min at room temperature (RT). Specimens
tography (MCAC): empty SPE cartridge (5 ml) was stoppered
were then rinsed in the same buffer (four times for 15 min)
at the base with round glass fibres, filled with 1.0 ml chelating
and then dehydrated with increasing concentrations of acetone
sepharose resin and placed on a SPE vacuum extractor. After
(50%, 70%, 90%) twice for 10 min each. Subsequently, samples
washing with 5 ml HPLC grade water, the column was condi-
were gradually infiltrated with increasing concentrations of LR-
tioned with 1 ml 0.1 M copper sulphate solution and then with
White resin (30%, 60%, 100%; London Resin Co., Berkshire,
1 ml MeOH/H2O (1:1, v/v). Sample extract was then loaded
UK) mixed with acetone (90%) for a minimum of 3 h per step.
into the cartridge that was then washed with 3 ml MeOH/H2O
Samples were finally embedded in pure and fresh LR-White
and 2 ml H2O. Elution of analyte was achieved with 2 ml
resin and polymerised at 50 °C for 48 h under anaerobic condi-
McIlvaine solution, pH 4.5. The extract was dried under a
tions. Ultrathin sections (80 nm) were cut with a Reichert
stream of N2 and finally resuspended in 1 ml MeOH. HPLC-
Ultracut S ultramicrotome (Leica Microsystems, Vienna,
MS analysis was performed following the Dasenaki & Thomai-
Austria) and samples observed with transmission electron
dis (2010) modified method. Chromatographic analyses were
microscopy (CEM Zeiss 902) at 80 kV.
performed using an HPLC system with a LC-20AD pump, aCBM-20A controller, a SIL-20a HT auto-sampler and a diode
Immuno-gold labelling
array SPD –M20A (Shimadzu, Tokyo, Japan) with a mass spec-trometer. Separations were achieved using a Dr. Maisch
For TC subcellular localisation, immuno-gold labelling was
UHPLC reprosil-pr-ods-3 column (100 9 2 mm, 3 lm particle
performed according to Canini et al. (2004). Briefly, ultrathin
Plant Biology 2013 German Botanical Society and The Royal Botanical Society of the Netherlands
Di Marco, Gismondi, Canuti, Scimeca, Volpe & Canini
Tetracycline uptake and effects in plants
sections (70–80 nm) were included in LR-White resin, as
and then absorbance was measured at 415 nm with spectro-
described before, and then assembled on nickel with formvar
photometer (Uvikon 860 UV-visible; Kontron). A calibration
grids. Samples were pre-incubated for 5 min in 1% H2O2,
washed for 5 min in 0.1% PBS Tween and incubated 30 min in
(10–250 lgml 1) in methanol. Total flavonoid amount is
5% BSA in 0.1% PBS Tween. Then, sections were rinsing in
expressed in milligram quercetin equivalent per gram sample
0.1% PBS Tween and incubated overnight with a primary anti-
fresh weight (mgQEg 1 FW). Total phenols were determined
body (polyclonal from host rabbit anti-TC, conjugated with
with the Folin Ciocalteu reagent (McDonald et al. 2001;
10-nm gold particles, 1:20 dilution in 1% BSA; Antibodies on
Gismondi et al. 2012). Plant extract of 0.5 ml was mixed with
line, Georgia, USA). Observations were carried out with a Zeiss
5 ml Folin Ciocalteu reagent (1:10 v/v in distilled water) and
CEM 902 electron microscope at 80 kV. The labelling was car-
4 ml1 M Na2CO3. The mixture was incubated for 60 min and
ried out according to the method described in Canini et al.
total phenols determined with colorimetric at 765 nm (Uvikon
(1992) and Taylor et al. (1994).
860 UV-visible; Kontron). A standard curve was preparedusing 0–250 mgl 1 solutions of gallic acid in water. Totalphenols were expressed in milligram gallic acid equivalent per
Biometric analysis
gram sample fresh weight (mgGAEg 1 FW).
Control and TC-treated plants (20 for each treatment) wereanalysed for germination, root and shoot length, leaf length
Western blot analysis
and width and total length. Percentage germination was calcu-lated as the number of seeds that germinated per flask with
Plant protein extraction was performed according to Wang
respect to the total number of sown seeds 9 100. Primary root
et al. (2005). Sample of 500 mg was powdered with a mortar
length was measured from the tip of the primary root to the
and pestle under liquid N2. All steps were performed on ice
hypocotyl, while secondary root length was measured from the
and solvents were used cold. The sample was resuspended in
tip of the secondary root to the beginning of the primary root.
2 ml 100% acetone vortexed and centrifuged at 16,000 g for
Leaf length was determined from the beginning of the leafstalk
3 min. The pellet was washed consecutively with: 2 ml 100%
to the tip of the leaf, and width from the upper to the lower
acetone, 2 ml 10% acetone, twice with 2 ml 10% TCA and
side. Total length was measured from the tip of the primary
twice with 2 ml 80% acetone. Finally, it was resuspended with
root to the tip of the shoot. All measurements were obtained
0.8 ml phenol (Tris-buffered, pH 8.0) and 0.8 ml SDS buffer
using a gauge and are indicated in cm. Shoot length was deter-
(30% sucrose, 2% SDS, 0.1 M Tris-HCl pH 8.0, 5% 2-mercap-
mined by subtracting root length from total length. On the
toethanol). After centrifugation at 16,000 g for 3 min, the phe-
basis of these results, for each sample was also estimated effec-
nolic portion was recovered and added to 4 ml 100% methanol
tive concentration (EC) for EC 25 and EC 50: the minimum
and 0.1 M ammonium acetate. Sample was frozen 30 min at
amount of antibiotic (mgl 1) able to inhibit plant growth of
20 °C and then centrifuged at 16,000 g for 15 min. The new
50% and 25%, with respect to the control, as reported in
pellet was washed twice with 2 ml 0.1 M ammonium acetate
Baguer et al. (2000).
and twice with 2 ml 80% acetone. The pellet was dried, dis-solved in 25 ll lysis buffer (7 M urea, 2 M thiourea, 4% 3-[(3-cholamido propyl)-dimethylammonio]-1-propane sulphonate
Photosynthetic pigment content
(CHAPS), 0.8% IPG-buffer pI 3–10 NL, 1% DTT) and centri-
Photosynthetic pigments were extracted from plant material in
fuged at 16,000 g for 30 min at 18 °C. The supernatant was
80% acetone for 24 h in darkness at 4 °C. The resulting suspen-
recovered and stored at
20 °C. Protein concentration was
sion was centrifuged for 5 min at 3000 g and absorbance of
quantified by the Bradford method (Bradford 1976), using
the supernatant measured at 470, 645 and 663 nm with an
bovine serum albumin as standard. Samples were then sepa-
UV/Vis spectrophotometer (Uvikon 860 UV-visible; Kontron,
rated on 12% SDS polyacrylamide gel, transferred onto nitro-
Eching, Germany). Pigment levels were calculated from an
cellulose Protran membrane (Schleicher and Schuell) and
equation allowing a simultaneous determination, in the same
incubated with the following primary antibodies: rabbit poly-
sample, of chlorophyll a (Chl a), b (Chl b) and carotenoids
clonal anti-b-actin, goat polyclonal anti-superoxide dismutase
(Car), as previously described (Lichtenthaler 1987). Results
1 (SOD1) and mouse monoclonal anti-catalase (CAT) (Sigma-
were expressed in microgram pigment per gram sample fresh
Aldrich). Primary antibodies were revealed using horseradish
weight (lgg 1 FW).
peroxidase-conjugated donkey anti-goat, goat anti-rabbit andanti-mouse antibodies (Jackson Immunoresearch) and the ECLchemiluminescence detection system (Pierce). Molecular
Flavonoid and total phenol content
weight (MW1 Pierce, MW2 Fermentas) was obtained by white
Total flavonoid and phenol concentrations were measured fol-
light scanning. SOD1 (18 kDa) and CAT (64 kDa) standards
lowing a modified Pourmorad et al. (2006) method. A total of
were purchased from Sigma-Aldrich. Quantifications were per-
1 g of each sample was powdered and dissolved in 10 ml meth-
formed eith the LAS3000 Image System (Fuji) and IMAGE QUANT
anol for 48 h. After that, the solvent was removed under a
software (GE Healthcare, Milan, Italy).
stream of N2 and finally resuspended in 1 ml methanol. For fla-vonoid determination was used the aluminium chloride colori-
metric method (Chang et al. 2002): 0.5 ml plant extract wasadded to 0.1 ml 10% aluminium chloride, 0.1 ml1 M potas-
Crude protein solutions were extracted by homogenising plants
sium acetate, 1.5 ml methanol and 2.8 ml distilled water. The
with a mortar and pestle under liquid N2. A total of 1 g crushed
reaction mixture remained at room temperature for 30 min
sample was dissolved in 10 ml phosphate buffer (0.05 M PBS,
Plant Biology 2013 German Botanical Society and The Royal Botanical Society of the Netherlands
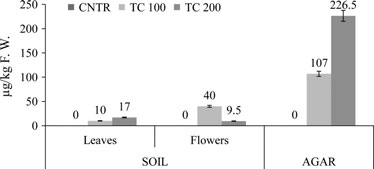
Tetracycline uptake and effects in plants
Di Marco, Gismondi, Canuti, Scimeca, Volpe & Canini
1% polyvinylpyrollidone, pH 7.8). After filtration through four
17.00 lgkg 1 in leaves, and 40.00 and 9.50 lgkg 1 in flowers.
layers of cheesecloth to remove debris, the homogenate was
In contrast, seedlings grown in agar had higher levels of TC:
centrifuged at 10,000 g for 10 min. All operations were
107.00 lgkg 1 and 226.50 lgkg 1, respectively, for 100 and
performed at 4 °C. The supernatant was used for enzyme activ-
200 mgl 1 TC treatments.
ity, as described in Polle et al. (1997). SOD1 activity was mea-sured on the basis of ability to inhibit photochemical reduction
Confocal microscopy analysis
of nitro-blue tetrazolium (Masia 1998). The reaction mixturecontained 50 mM phosphate buffer (pH 7.8), 13 mM methio-
Tetracycline-treated plant samples grown in agar were stud-
nine, 75 lM nitro-blue tetrazolium, 2 lM riboflavin, 100 mM
ied with CM to investigate and localise antibiotic accumula-
EDTA and 0–200 ll plant extract. The reaction mixture was
tion sites. Figure 2 contains images of root (A and D), stem
read at 560 nm using a UV-Vis spectrophotometer (Uvikon
(B and E) and leaf (C and F) portions of treated plants with
860 UV-visible; Kontron). One unit of SOD1 activity was
TC 100 mgl 1 (A, B and C) and 200 mgl 1 (D, E and F)
defined as the amount of enzyme that caused 50% inhibition
for 30 days. The clear presence of yellow colour denotes the
of the initial rate of the reaction in the absence of enzyme. The
presence of TC throughout the plant. More TC was detected
activity of SOD1 was expressed as unit per gram sample fresh
after the higher dose than the lower dose. In all cases, TC
weight (Ug 1 FW). CAT activity was calculated as reported in
was everywhere in the sections, but especially around the
Aebi (1984). The reaction mixture contained 10 mM H2O2,
cells. Special hotspots were stomatal cells with 200 mgl 1
50 mM K3PO4 buffer, pH 7, and 800 ll extract in a total
TC (F). Figure S1 shows a 3-D reconstruction of TC (yellow)
volume of 1.2 ml. The principle of this test was based on
and Chl (red) disposition in stems (A) and leaves (B) of a
hydrolysis of H2O2 and the relative decrease in absorbance at
plant exposed to high doses of TC. In particular, in leaves
240 nm. A reduction in absorbance of 0.01 at 240 nm in 1 min
(B) it was clear that Chl dissipated inside cells with respect
was considered as one unit enzyme activity. CAT activity
to TC, which accumulated in apoplastic spaces and in stoma-
was expressed as unit per minute per gram fresh weight
tal cells (Fig. 2F). In control plants no TC signal was detected
(Umin 1 g 1 FW).
(data not shown).
Statistical analysis
Transmission electron microscopy observations
All experiments were repeated in triplicate. Results are shown
Transmission electron microscope analysis was performed
as mean SD of three independent measurements. Analysis of
to examine whether TC distribution in seedlings was not
variance was conducted using one-way ANOVA with the statisti-
confined to the apoplastic space, as demonstrated in CM
cal software PAST 0.45 (PAlaeontological STatistics).
observations. As shown in Fig. 3, gold particles associatedwith the TC antibody were clearly visible as black spots,both in cell cytoplasm and in mitochondria, even if at low
Tetracycline uptake
Biometric analysis
Iberis sempervirens were grown in soil and in agar in the pres-ence of TC 100 and 200 mgl 1, as described in Material and
To study the effects of TC on germination and development,
Methods, in order to determine their antibiotic absorption
I. sempervirens seeds were grown under controlled conditions
capacity. With respect to the control, where we did not find
in agar, with or without TC (TC 100 mgl 1, TC 200 mgl 1
antibiotic traces, the extracts of all treated plants showed TC
and control). Figure S2 shows percentage germination of con-
presence. The amount of TC detected in each sample is
trol and treated seeds. TC affected seed germination in a con-
reported in Fig. 1. We separately analysed the level of TC in
centration-dependent manner: after 10, 20 and 30 days of
flowers and leaves and also in whole seedlings grown in agar.
treatment, compared to the control, TC 100 mgl 1 inhibited
In soil, for both treatments (TC 100 and 200 mgl 1), TC accu-
germination of 20.1, 8.5 and 6.1%, while TC 200 mgl 1
mulation in adult plants was, respectively, 10.00 and
decreased this percentage to 15.1, 35.8 and 23.8%. The mor-phology of seedlings grown in agar for 30 days in the absenceof antibiotic or in the presence of TC 100 mgl 1 and TC200 mgl 1 are shown, respectively, in Figure S3 A, B and C.
With respect to the control, TC treatments altered the growthand development of seedlings: total length of control, TC 100and
1.45 0.10 cm and 1.50 0.10 cm; primary root length was5.80 0.25, 0.25 0.10 and 0.27 0.05 cm; shoot length was5.67 0.60, 1.20 0.10 and 1.23 0.10 cm, cotyledon lengthwas 0.73 0.10, 0.50 0.05 and 0.57 0.10 cm; and cotyle-don width was 0.53 0.10, 0.42 0.05 and 0.40 0.05 cm.
Fig. 1. Detection of TC in different parts (leaves and flowers) of plants
Secondary roots and secondary leaves did not develop in trea-
grown in soil and in seedlings cultivated in agar. Results are expressed as
ted plants (Table 1). Effective antibiotic concentrations able to
lgTCkg 1 FW. Data are reported as mean of three different experi-
inhibit growth 25% and 50% (EC 25 and EC 50), with respect
to the control, were determined (Table 2).
Plant Biology 2013 German Botanical Society and The Royal Botanical Society of the Netherlands
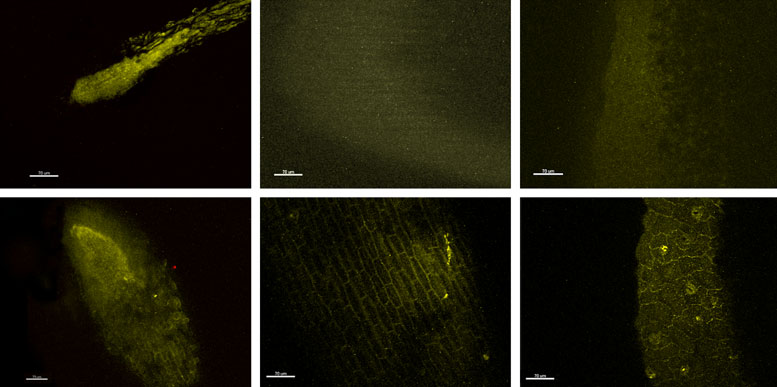
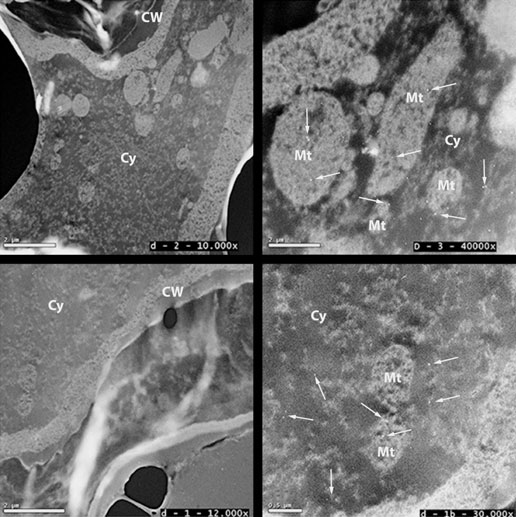
Di Marco, Gismondi, Canuti, Scimeca, Volpe & Canini
Tetracycline uptake and effects in plants
Fig. 2. Apoplastic TC distribution in seedlings grown in agar. CM analysis showed root (A), stem (B), and leaf (C) images of plants treated with 100 mg/l ofautoflorescent antibiotic. Root (D), stem (E) and leaf (F) districts of seedlings exposed to 200 mgl of TC were also reported. White bars below images corre-spond to 70 lm.
Fig. 3. Observations with TEM of TC subcellular localisa-tion in treated plant cotyledons (A and C). Detectionwas performed with an immunogold-labelled antibodydirected against TC. Arrows indicate position of someTC molecules (black spots) in cells. Black bars indicateunit of the images in micrometer and in white squaresare reported the magnification. B and D are, respec-tively, the enlargements of A and C. Cy, cytoplasm, Mt,mitochondrion, CW, cell wall.
biotic treatment (Table 3). In comparison to the control, TC
Primary and secondary metabolite content
100 and TC 200 mgl 1 samples, respectively, presented a
The influence of TC on plant production of photosynthetic
reduction of 23.6% and 44.5% Chl a, 30.4% and 47.7% Chl
pigments and secondary metabolites was analysed. The con-
b and a loss in Car of 18.6% and 42.2%. Levels of flavo-
tent of Chl a, b and Car was measured in plants after anti-
noids evaluated in leaf extracts (Fig. 4A), with respect to the
Plant Biology 2013 German Botanical Society and The Royal Botanical Society of the Netherlands
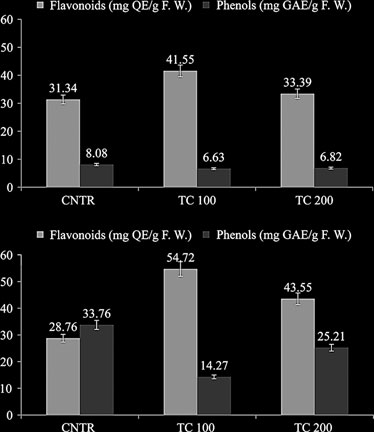
Tetracycline uptake and effects in plants
Di Marco, Gismondi, Canuti, Scimeca, Volpe & Canini
Table 1. Biometric analysis: total length (TL), primary (PRL) and secondary (SRL) root length, shoot length (SL), secondary leaf number (SLN), cotyledon length(CL) and width (CW) are reported for control (CNTR) and treated (TC 100 and TC 200) seedlings grown in agar. Results were expressed in cm as the mean sdof three different measurements. All observed differences were statistically significant (ANOVA, TC 100: F = 5.79, P < 0.05; TC 200: F = 5.71, P < 0.05).
biometric parameter
Table 2. Effective TC concentrations (EC) that cause 25% and 50% plant
growth reduction compared to the control. Data are reported in mgl 1.
Results are expressed in cm as mean SD of different results.
TC concentration (mgl 1)
Table 3. Photosynthetic pigment content, quantified in Iberis sempervirensplants treated with TC (TC 100 and TC 200) or not (CNTR) for 30 days,expressed in lgg 1 FW. Results are reported as mean SD of three inde-pendent experiments. All differences were statistically significant (ANOVA,F = 6.59, P < 0.01).
pigments (lgg 1 FW)
Fig. 4. (A) Flavonoid and phenol content in leaf extracts of Iberis sempervi-rens grown in soil. Results are mg standard per g sample fresh weight(mgQEg 1 FW; mgGAEg 1 FW). Data are as means SD of three differ-
control, increased by 32.5% and 6.5%, respectively, after
ent experiments. All values are significantly different at P < 0.01, F = 18.31.
treatment with low (TC 100) and high (TC 200) doses of
(B) Flavonoid and phenol content in flower extracts of Iberis sempervirens
antibiotic for 30 days. However, phenols decreased following
grown in soil. Results are mg standard per g sample fresh weight
both TC treatments: 17.9% (TC 100) and 15.6% (TC 200),
(mgQEg 1 FW; mgGAEg 1 FW). Data are means SD of three different
compared to the control. Secondary metabolite content was
experiments. All values were significantly different at P < 0.01, F = 14.45.
also measured in flower samples (Fig. 4B): flavonoids wereenhanced 90.2% (TC 100) and 51.4% (TC 200), with
respectively, but TC 200 extracts had lower enhancement of
respect to the control, while total phenolic synthesis was
these enzymes of, respectively, 96.26% and 36.03%, with
inhibited 57.7% (TC 100) and 25.3% (TC 200), compared
respect to the control (Fig. 5D). Although the antibodies
to control extracts.
used in Western blotting were extracted from animals theyalso showed high specificity for plant protein extracts. Inparticular, full gels are reported in Figure S4 to confirm
Antioxidant enzyme level and activity
the specificity and molecular size of the detected proteins
Cell antioxidant enzymes, SOD and CAT, were quantified
and their standards. To support these data, SOD1 and CAT
with Western blot analysis (Fig. 5A, B). In leaf extracts,
activity were also measured in leaf extracts using spectro-
with respect to the control, SOD1 and CAT increased of
photometry (Fig. 6). After treatment with low doses of TC
266.07 and 148.9%, respectively, in the TC 100 sample;
(100 mgl 1), SOD1 and CAT had increased activity, respec-
while in TC 200 plants only CAT was up-regulated
of 156.07 3.90 Umg 1 FW and 17.93 1.15
(30.77%) but SOD1 decreased to 31.87%, compared to
Umin 1 g 1 FW. At high doses of TC (200 mgl 1), SOD
control plants (Fig. 5C). In flowers, TC 100-treated plants
activity was 98.26 2.46 Umg 1 FW and CAT 13.45
increased SOD1 and CAT levels of 202.78% and 38.61%,
0.52 Umin 1 g 1 FW.
Plant Biology 2013 German Botanical Society and The Royal Botanical Society of the Netherlands
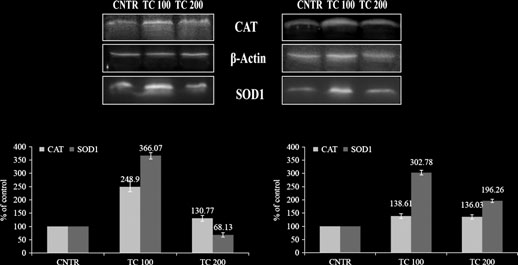
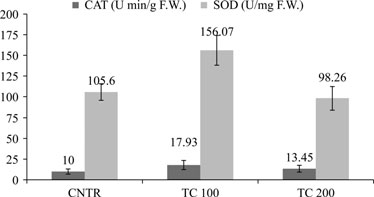
Di Marco, Gismondi, Canuti, Scimeca, Volpe & Canini
Tetracycline uptake and effects in plants
Fig. 5. Immunoblot analysis of CAT and SOD1 protein
levels. A (leaf protein extract) and B (flower proteinextract) are representative immunoblots of the threeindependent experiments. b-actin was used as loadingcontrol. Results are expressed as fold changes withrespect to the control (C, leaf extract; D, flower extract).
Data are mean of three different experiments SD (inFigure S4 full gels are also reported with MW and con-trol standards).
sent an alarm for human health: in fact, through this route notonly animal origin foods but also vegetables can be consideredantibiotic sources (Dolliver et al. 2007). To investigate antibi-otic diffusion modality and to localise the principal accumula-tion sites in plants, we studied TC-treated seedlings grown inagar using CM and TEM. The CM analysis was possible thanksto TC natural fluorescence. In all plant portions (root, stemand leaf; Fig. 2, Figure S1), we detected antibiotic moleculeseverywhere in the apoplastic space. TC signal was rarely presentin cells, except in particular hotspots such as inside stomatalcells (Fig. 2F). In these cells the high turnover of water, due toconservation of turgor pressure, might cause accumulation of
Fig. 6. Activity of CAT and SOD1 in leaf extracts of plants grown in soil,
the antibiotic. These observations clearly demonstrated that TC
with or without TC, in units per minute per g sample fresh weight
entered and propagated in plants through apoplastic transport.
(Uming 1 FW) and units per mg sample fresh (Umg 1 FW), respectively.
Therefore, the present work represents an important study in
Results are means of three different experiments SD.
plant biology, as many other studies only verified and demon-strated antibiotic uptake in plants but none clarified themodality (Migliore et al. 2003; Boonsaner & Hawker 2012). InTEM analysis (Fig. 3), however, TC labelled with a golden anti-
body was also detected within cells, supporting the possibility
Recently, antibiotics were considered an emerging group of
that a small amount of TC molecules were transported into the
environmental contaminants because of their negative poten-
cytoplasm and even inside mitochondria.
tial effects on human health and ecological communities
Tetracycline is a bacteriostatic agent that limits the growth
(Dirany et al. 2011). Antibiotics are widely used to treat infec-
of bacteria by interfering with protein synthesis; it binds the
tious diseases in both humans and animals, and to promote
prokaryote small ribosomal subunit (30S) and inhibits amino-
livestock farming, aquaculture and agriculture (Gao et al.
acyl-tRNA access to the ribosomal acceptor A site (Orth et al.
2012). Many antibiotics cannot be completely absorbed or me-
2000; Pioletti et al. 2001). For these reasons, the TC amount
tabolised and most are introduced, through excretion, into the
accumulated in mitochondria could be explained by activity of
sewage system, which has become an important source of anti-
this molecule against 70S ribosomes that they contain. New
biotics in the environment (Leung et al. 2012). It has reported
studies should be performed to clarify further TC transport
that plants grown on contaminated soil absorb antibiotics (Hil-
inside cells but, according to its action in bacteria (Nelson et al.
lis et al. 2010); therefore, aim of this work was the study antibi-
2003), we supposed that TC crossed the membrane through
otic uptake in I. sempervirens, principal bioaccumulation sites
active transport, not through the aquaporins (Kong et al.
and plant responses to high (200 mgl 1) and low (100 mgl 1)
2007). This research also suggested the suitability of TC for
doses of TC.
study of antibiotic absorption in plants because of its fluores-
Antibiotic uptake in plants, grown on contaminated soil or
cent properties and efficient uptake and transport from roots
agar, was investigated through chromatographic analysis
(Fig. 1). In all parts (leaves and flowers) of treated plants we
The last part of our work was focused on the TC treatment
found TC traces, in a dose-dependent manner. Absorption was
effects on plant phenotype and physiology. We observed that
very significant in plants grown in agar and not negligible in
TC inhibits seed germination (Figure S2). Biometric analysis
samples from treated soil. These differences might be due to
on seedlings grown in agar revealed that TC-treated plants had
the biotic soil component that is able both to degrade and to
a large reduction in growth, they lacked secondary roots, and
make TC less available to plants (Jensen 2001). Our results
leaves had decreased cotyledon length and width (Table 1).
confirmed plant ability to take up TC but, above all, they repre-
The EC50 and EC25 indicated that the development of treated
Plant Biology 2013 German Botanical Society and The Royal Botanical Society of the Netherlands
Tetracycline uptake and effects in plants
Di Marco, Gismondi, Canuti, Scimeca, Volpe & Canini
plant roots and stems was inhibited even at low doses of TC,
In conclusion, this research demonstrated that: (i) I. semper-
while cotyledons were less sensitive (Table 2), probably because
virens plants absorb TC from soil and agar via apoplastic trans-
roots were the direct organs in contact with the polluted med-
port; (ii) only a little amount of TC is internalised in cells,
ium (Migliore et al. 2003). Inhibition of mitochondrial and
where it probably inhibits mitochondrial ribosome activity;
plastid protein synthesis by TC is the principal cause of phyto-
(iii) TC caused a reduction of plant growth and photosynthetic
toxicity in treated plants (Bradel et al. 2000; Kasai et al. 2004);
activity; and (iv) plant cells try to balance the oxidative stress
therefore, we analysed if the stress induced by TC could modify
of TC by increasing the amount of antioxidant compounds,
plant photosynthetic activity. Chlorophyll a, b and carotenoid
such as flavonoids, and the levels and activity of some endoge-
content decreased in all treated plants (Table 3), suggesting a
nous antioxidant enzymes.
decrease in chloroplast photosynthetic efficiency, as easilyobserved in the chlorotic phenotype of some treated plant
leaves. We also observed that seedlings grown in agar in thepresence of TC were etiolated (Figure S3); the low levels of
We thank Roberto Targa for technical support, the Center of
photosynthetic pigments indicated the toxic nature of TC for
Advanced Microscopy ‘P.B. Albertano' of the Department of
plants (Murata et al. 2007).
Biology, University of Rome ‘Tor Vergata' and Dr. Elena
Secondary metabolites are a group of phenolic compounds
Romano for assistance in confocal microscopy analysis.
with known properties, which include free radical scavenging,inhibition of hydrolytic and oxidative enzymes and anti-inflam-
SUPPORTING INFORMATION
matory action (Gryglewski et al. 1987; Frankel 1995; Cook &Samman 1996). The amount of these compounds was reported
Additional Supporting Information may be found in the online
to increase in plants under stress in order to protect cell vitality
version of this article:
(Huseynova 2012). As expected, we found that TC treatment
Figure S1. Merged image of tetracycline (yellow) and chlo-
increased flavonoid levels in all parts of the plants but, surpris-
rophyll (red) content revealed with CM in seedling stems trea-
ingly, it reduced the simple phenol content (Fig. 4). This differ-
ted with 200 mgl 1 (A). 3-D reconstruction of tetracycline
ence might be associated with a specific inhibitory effect that TC
(yellow) and chlorophyll (red) disposition in a leaf of a seedling
could exercise on secondary metabolite synthetic pathways or
grown in 200 mgl 1 TC. White bar below the picture corre-
on their degradation (Arbona et al. 2013). Oxidations and
sponds to 70 lm.
reductions are essential processes for cell survival, but when
Figure S2. Percentage germination at 10, 20 and 30 days of
their equilibrium is modified, cellular stability is damaged; this
control and treated (TC 100 and 200 mgl 1) seed with respect
condition is known as oxidative stress. Plant exposure to envi-
to total planted seeds in agar.
ronmental contaminants (e.g. salts, pesticides, drugs, drought)
Figure S3. Images of seedlings grown in agar for 30 days in
leads to this altered condition (Schwanz & Polle 2001; Shigeoka
the absence (A) or with TC 100 mgl 1 (B) and 200 mgl 1
et al. 2002; Vranova et al. 2002); therefore, the last step of our
(C). Black bar corresponds to 1 cm.
work was the study of oxidative stress that TC induces in plant
Figure S4. Representative
cells. Western blot analysis was performed on leaf and flower
chemiluminescence) reported in full form. The specificity of
protein extracts in order to determine SOD1 and CAT quantity,
anti-CAT (64 kDa), anti-SOD1 (18 kDa) and anti-b-actin
as the principal cellular endogenous antioxidant enzymes
(42 kDa) antibodies in all protein extracts (leaf in upper
(Fig. 5, Figure S4). The activity of these proteins was also evalu-
panel and flower in lower panel) were confirmed from the
ated using spectrophotometry (Fig. 6). The results obtained
molecular weights. (acquired from white light scanning).
showed that both enzymes increased in level and activity, espe-
Commercial CAT and SOD1 standards were also loaded
cially after low doses of TC. All these data confirm that TC
into a polyacrylamide gel and detected with the same anti-
causes oxidative stress in plants and that cells try to limit it using
bodies as plant extracts to confirm their specificity (on right
antioxidant enzymes and molecules.
in lower panel).
Bradford M.M. (1976) A rapid and sensitive method
Behavior of pharmaceuticals, cosmetics and hor-
for the quantitation of microgram quantities of pro-
mones in a sewage treatment plant. Water Research,
Aebi H. (1984) Catalase in vitro. Methods in Enzymol-
tein utilizing the principle of protein–dye binding.
38, 2918–2926.
ogy, 105, 121–126.
Analytical Biochemistry, 72, 248–254.
Chang C., Yang M., Wen H., Chern J. (2002) Estima-
Arbona V., Manzi M., de Ollas C., Gomez-Cadenas A.
Brambilla G., Patrizii M., De Filippis S.P., Bonazzi G.,
tion of total flavonoid content in propolis by two
(2013) Metabolomics as a tool to investigate abiotic
Mantovi P., Barchi D., Migliore L. (2007) Oxytetra-
complementary colorimetric methods. Journal of
stress tolerance in plants. A review. International
cycline as environmental contaminant in arable
Food and Drug Analysis, 10, 178–182.
Journal of Molecular Sciences, 14, 4885–4911.
lands. Analytica Chimica Acta, 586, 326–329.
Cook N.C., Samman S. (1996) Flavonoids – chemistry,
Baguer A.J., Jensen J., Krogh P.H. (2000) Effects of
Canini A., Civitareale P., Marini S., Grilli Caiola M., Rot-
metabolism, cardioprotective effects, and dietary
antibiotics oxytetracycline and tylosin on soil fauna.
ilio G. (1992) Purification of iron superoxide dismu-
sources. The Journal of Nutritional Biochemistry, 7,
Chemosphere, 40, 751–757.
tase from the cyanobacterium Anabaena cylindrica
Boonsaner D., Hawker D.W. (2012) Investigation of
Lemm. and localization of the enzyme in heterocysts
Cox G., Wright G.D. (2013) Intrinsic antibiotic resis-
the mechanism of uptake and accumulation of zwit-
by immunogold labelling. Planta, 187, 438–444.
tance: mechanisms, origins, challenges and solutions.
terionic tetracyclines by rice (Oryza sativa L.). Eco-
Canini A., Giovinazzi J., Iacovacci P., Pini C., Grilli
International Journal of Medical Microbiology, 303,
toxicology and Environmental Safety, 78, 142–147.
Caiola M. (2004) Localization of a carbohydrate epi-
Bradel B.G., Preil W., Jeske H. (2000) Remission of the
tope recognised by human IgE in pollen of Cupress-
Dasenaki M.E., Thomaidis N.S. (2010) Multi-residue
free-branching pattern of Euphorbia pulcherrima by
aceae. Journal of Plant Research, 117, 147–153.
determination of seventeen sulfonamides and five
tetracycline treatment. Journal of Phytopathology,
Carballa M., Omil F., Lema J.M., Liompart M., Garcia-
tetracyclines in fish tissue using a multi-stage
148, 587–590.
Jares C., Rodriguez I., Gomez M., Ternes T. (2004)
LC–ESI–MS/MS approach based on advanced mass
Plant Biology 2013 German Botanical Society and The Royal Botanical Society of the Netherlands
Di Marco, Gismondi, Canuti, Scimeca, Volpe & Canini
Tetracycline uptake and effects in plants
spectrometric techniques. Analytica Chimica Acta,
Kapustka L.A. (1997) Selection of phytotoxicity tests
Orth P., Schnappinger D., Hillen W., Saenger W.,
672, 93–102.
for use in ecological risk assessments. In: Wang W.,
Hinrichs W. (2000) Structural basis of gene
Di Marco G., Canuti L., Impei S., Leonardi D., Canini A.
Gorsuch J.W., Hughes J.S. (Eds), Plants for environ-
(2012) Nutraceutical properties of honey and pollen
mental studies. Lewis, CRC Press, Boca Raton, FL,
repressor-operator system. Nature Structural Biol-
produced in a natural park. Agricultural Sciences, 3,
USA, pp 515–548.
ogy, 7, 215–219.
Kasai K., Kanno T., Endo Y., Wakasa K., Tozawa Y.
Pautke C., Vogt S., Kreutzer K., Haczek C., Wexel G.,
Dirany A., Aaron S.E., Oturan N., Sires I., Oturan
(2004) Guanosine tetra- and pentaphosphate syn-
Kolk A., Imhoff A.B., Zitzelsberger H., Milz S.,
M.A., Aaron J.J. (2011) Study of the toxicity of sulfa-
thase activity in chloroplasts of a higher plant: asso-
Tischer T. (2010) Characterization of eight different
methoxazole and its degradation products in water
ciation with 70S ribosomes and inhibition by
tetracyclines: advances in fluorescence bone labeling.
by a bioluminescence method during application of
tetracycline. Nucleic Acids Research, 32, 5732–5741.
Journal of Anatomy, 217, 76–82.
the electro-Fenton treatment. Analytical and Bioana-
Kolpin D.W., Furlong E.T., Meyer M.T., Thurman
Pioletti M., Schlunzen F., Harms J., Zarivach R., Gluh-
lytical Chemistry, 400, 353–360.
E.M., Zaugg S.D., Barber L.B., Buxton H.T. (2002)
mann M., Avila H., Bashan A., Bartels H., Auerbach
Dolliver H., Kumar K., Gupta S. (2007) Sulphameth-
Pharmaceuticals, hormones, and other organic
T., Jacobi C., Hartsch T., Yonath A., Franceschi F.
azine uptake by plants from manure-amended soil.
wastewater contaminants in U.S. streams, 1999–
(2001) Crystal structures of complexes of the small
Journal of Environmental Quality, 36, 1224–1230.
2000. A national reconnaissance. Environmental
ribosomal subunit with tetracycline, edeine and IF3.
Frankel E. (1995) Nutritional benefits of flavonoids.
Science and Technology, 36, 1202–1211.
EMBO Journal, 20, 1829–1839.
International conference on food factors: Chemistry
Kong W.D., Zhu Y.G., Liang Y.C., Zhang J., Smith
Polle A., Eiblmeier M., Sheppard L., Murray M. (1997)
F.A., Yang M. (2007) Uptake of oxytetracycline and
Responses of antioxidative enzymes to elevated CO2
Abstracts, C6- 2.
its phytotoxicity to alfalfa (Medicago sativa L.).
in leaves of beech (Fagus sylvatica L.) seedlings
Gao L., Shi Y., Li W., Niu H., Liu J., Cai Y. (2012)
Environmental Pollution, 147, 187–193.
grown under a range of nutrient regimes. Plant, Cell
Occurrence of antibiotics in eight sewage treat-
Kumar K., Gupta S.C., Baidoo S.K., Chander Y., Rosen
& Environment, 20, 1317–1321.
ment plants in Beijing, China. Chemosphere, 86,
C.J. (2005a) Antibiotic uptake by plants from soil
Pourmorad F., Hosseinimehr S.J., Shahabimajd N.
fertilized with animal manure. Journal of Environ-
(2006) Antioxidant activity, phenol and flavonoid
Gismondi A., Serio M., Canuti L., Canini A. (2012)
mental Quality, 34, 2082–2085.
contents of some selected Iranian medicinal plants.
Biochemical, antioxidant and antineoplastic proper-
Kumar K., Gupta S.C., Baidoo S.K., Chander Y., Singh
African Journal of Biotechnology, 5, 1142–1145.
ties of Italian saffron (Crocus sativus L.). American
A.K. (2005b) Antibiotic use in agriculture and its
Schwanz P., Polle A. (2001) Differential stress
Journal of Plant Sciences, 3, 1573–1580.
impact on the terrestrial environment. Advances in
responses of antioxidative systems to drought in
Glassmeyer S.T., Furlong E.T., Kolpin D.W., Cahill
Agronomy, 87, 1–54.
pendunculate oak (Quercus robur) and maritime
J.D., Zaugg S.D., Werner S.L., Meyer M.T., Kryak
Leung H.W., Minh T.B., Murphy M.B., Lam J.C.W., So
pine (Pinus pinaster) grown under high CO2 concen-
D.D. (2005) Transport of chemical and microbial
M.K., Martin M., Lam P.K.S., Richardson B.J.
trations. Journal of Experimental Botany, 52, 133–
compounds from known wastewater discharges:
(2012) Distribution, fate and risk assessment of anti-
potential for use as indicators of human fecal con-
biotics in sewage treatment plants in Hong Kong,
Shigeoka S., Ishikawa T., Tamoi M., Miyagawa Y.,
tamination. Environmental Science & Technology, 39,
South China. Environment International, 42, 1–9.
Takeda T., Yabuta Y., Yoshimura K. (2002) Regula-
Lichtenthaler H.K. (1987) Chlorophylls and carote-
Gryglewski R.J., Korbut R., Robak J. (1987) On the
noids: pigments of photosynthetic biomembranes.
isoenzymes. Journal of Experimental Botany, 53,
mechanism of antithrombotic action of flavonoids.
Methods in Enzymology, 148, 350–382.
Biochemical Pharmacology, 36, 317–321.
Masia A. (1998) Superoxide dismutase and catalase
Sungpyo K., Aga D.S. (2007) Potential ecological and
Halling-Sørensen B.S., Nors Nielsen P.F., Lanzky F.,
activities in apple fruit during ripening and post-
human health impacts of antibiotics and antibiotic-
Ingerslev H.C., Holten L., Jorgensen S.E. (1998)
harvest and with special reference to ethylene. Physi-
resistant bacteria from wastewater treatment plants.
Occurrence, fate and effects of pharmaceutical sub-
ologia Plantarum, 104, 668–672.
Journal of Toxicology and Environmental Health. Part
stances in the environment. A review. Chemosphere,
McDonald S., Prenzler P.D., Autolovic M., Robards K.
B, Critical Reviews, 10, 559–573.
36, 357–393.
(2001) Phenolic content and antioxidant activity of
Taylor P.E., Staff I.A., Singh M.B., Knox R.B. (1994)
Hillis D.G., Fletcher J., Keith R., Solomon K.R., Sibley
olive extracts. Food Chemistry, 73, 73–84.
Localization of the two major allergens in rye-grass
P.K. (2010) Effects of ten antibiotics on seed germi-
Migliore L., Brambilla G., Casoria P., Civitareale C.,
pollen using specific monoclonal antibodies and
nation and root elongation in three plant species.
Cozzolino S., Gaudio L. (1996) Effects of sulpha-
quantitative analysis of immunogold labelling.
dimethoxine contamination on barley (Hordeum
Histochemical Journal, 26, 392–398.
Toxicology, 60, 220–232.
distichum L., Poaceae, Liliopsida). Agriculture Ecosys-
Vranova E., Inze D., Van Breusegem F. (2002) Signal
Hirsch R., Ternes T., Haberer K., Kratz K.L. (1999)
tems & Environment, 60, 121–128.
transduction during oxidative stress. Journal of
Occurrence of antibiotics in the aquatic environ-
Migliore L., Cozzolino S., Fiori M. (2003) Phytotoxic-
Experimental Botany, 53, 1227–1236.
ment. Science of the Total Environment, 225,
ity to and uptake of enrofloxacin in crop plants.
Wang W., Vignani R., Scali M., Cresti M. (2005) A uni-
Chemosphere, 52, 1233–1244.
versal and rapid protocol for protein extraction from
Huseynova I.M. (2012) Photosynthetic characteristics
Migliore L., Godeas F., De Filippis S.P., Mantovi P.,
recalcitrant plant tissues for proteomic analysis. Elec-
and enzymatic antioxidant capacity of leaves from
Barchi D., Testa C., Rubattu N., Brambilla G. (2010)
trophoresis, 27, 2782–2786.
wheat cultivars exposed to drought. Biochimica et
Hormetic effect(s) of tetracyclines as environmental
Xue B., Zhang R., Wang Y., Liu X., Li J., Zhang G.
Biophysica Acta, 1817, 1516–1523.
contaminant on Zea mays. Environmental Pollution,
(2013) Antibiotic contamination in a typical devel-
Jensen J. (2001) Veterinary medicines and soil qual-
158, 129–134.
oping city in south China: occurrence and ecological
ity: ‘The Danish Situation as an Example'. In:
Murata N., Takahashi S., Nishiyama Y., Allakhverdiev
risks in the Yongjiang River impacted by tributary
Daughton C.G., Jones-Lepp T.L. (Eds), Scientific
S. (2007) Photoinhibition of photosystem II under
discharge and anthropogenic activities. Ecotoxicology
and regulatory issues, symposium series, 791. Amer-
environmental stress. Biochimica et Biophysica Acta,
and Environmental Safety, 92, 229–236.
ican Chemical Society Washington, DC, USA, pp
1767, 414–421.
Zuccato E., Castiglioni S., Fanelli R., Bagnati R. (2006)
Nelson M.L., Hillen W., Greenwald R.A. (2003) Tetra-
Pharmaceuticals in the environment in Italy: causes,
Jørgensen S.E., Halling-Sørensen B. (2000) Drugs in
cyclines in biology, chemistry and medicine. Chem-
occurrence, effects and control. A Review. Environ-
the environment. Chemosphere, 40, 691–699.
BioChem, 1, 235–236.
mental Science and Pollution Research, 13, 15–21.
Plant Biology 2013 German Botanical Society and The Royal Botanical Society of the Netherlands
Source: http://www.marcoinfussi.it/docs/bonifica.archivio/articoli.scientifici.archivio/Canini%20-%20Tetracycline%20accumulates%20in%20Iberis%20sempervirens.pdf
IEEE TRANSACTIONS ON INFORMATION THEORY, VOL. 56, NO. 9, SEPTEMBER 2010 Thinning, Entropy, and the Law of Thin Numbers Peter Harremoës, Member, IEEE, Oliver Johnson, and Ioannis Kontoyiannis, Senior Member, IEEE Abstract—Rényi's thinning operation on a discrete random vari- where the random variables are independent and identi-
Bol SCCALP 217 64p 21/9/11 17:03 Página 169 BOL PEDIATR 2011; 51: 169-176 Tratamiento combinado de la retención voluntaria de hecesmediante fármacos y terapia ocupacional I. BEAUDRY BELLEFEUILLE1, E. RAMOS POLO2 1Clínica de Terapia Ocupacional Pediátrica Beaudry-Bellefeuille. Oviedo. 2Pediatra-puericultor. Oviedo. fundizar y refinar los métodos de valoración y tratamien-to para esta población.