Qpcrupdate.eu2

Am J Physiol Endocrinol Metab 285: E252–E261, 2003.
First published April 1, 2003; 10.1152/ajpendo.00557.2002.
Effects of dexamethasone and colostrum intakeon the somatotropic axis in neonatal calves
Stephanie N. Sauter,1 Edgar Ontsouka,1 Bettina Roffler,1
Yolande Zbinden,1 Chantal Philipona,1 Michael Pfaffl,2
Bernhard H. Breier,3 Ju
¨ rg W. Blum,1 and Harald M. Hammon1
1Division of Animal Nutrition and Physiology, Faculty of Veterinary Medicine, Institute of AnimalGenetics, Nutrition, and Housing, University of Berne, CH-3012 Berne, Switzerland; 2Centre of Lifeand Food Sciences, Institute of Physiology, Weihenstephan, Technical University of Munich,D-85354 Freising-Weihenstephan, Germany; and 3Department of Pediatrics, Research Centrefor Developmental Medicine and Biology, University of Auckland, NZ-92019 Auckland, New Zealand
Submitted 19 December 2002; accepted in final form 28 March 2003
Sauter, Stephanie N., Edgar Ontsouka, Bettina Rof-
fetal to the postnatal status and function (7, 11, 19).
fler, Yolande Zbinden, Chantal Philipona, Michael
Cortisol stimulates hepatic GH receptor (GHR) and
Pfaffl, Bernhard H. Breier, Ju
¨ rg W. Blum, and Harald
insulin-like growth factor I (IGF-I) mRNA levels in the
M. Hammon. Effects of dexamethasone and colostrum in-
sheep fetus (30), and dexamethasone (Dexa) increases
take on the somatotropic axis in neonatal calves. Am J
hepatic GHR mRNA levels in rabbits (24). Studies with
Physiol Endocrinol Metab 285: E252–E261, 2003. First pub-
hepatocytes indicate an increase of GHR and IGF-I
lished April 1, 2003; 10.1152/ajpendo.00557.2002.—Gluco-corticoids and colostrum feeding influence postnatal matura-
expression and enhanced IGF-I response to GH after
tion of the somatotropic axis. We have tested the hypothesis
Dexa administration (5). In neonatal pigs, Dexa treat-
that dexamethasone (Dexa) affects the somatotropic axis in
ment increases postnatal growth and stimulates func-
neonatal calves dependent on colostrum intake. Calves were
tioning of the somatotropic axis immediately after
fed either with colostrum or with a milk-based formula (n ⫽
birth (10). However, findings concerning effects of glu-
14/group), and, in each feeding group, one-half of the calves
cocorticoids on the somatotropic axis are inconsistent,
were treated with Dexa (30 g 䡠 kg body wt⫺1 䡠 day⫺1). Pre-
perhaps because of different species or age (9, 38).
and postprandial blood samples were taken on days 1, 2, 4,
Cortisol reduces the prenatal growth rate in sheep
and 5, and liver samples were taken on day 5 of life. Dexa
fetus during late gestation (17), and glucocorticoids
increased insulin-like growth factor (IGF)-I, but decreased
inhibit postnatal growth, in part, by direct interaction
growth hormone (GH) and IGF-binding protein (IGFBP)-1
with the somatotropic axis (9, 48, 49). Obviously, the
and -2 plasma concentrations and increased GH receptor(GHR) mRNA levels in liver. Dexa increased IGF-I mRNA
gestational stage and the time around birth modify
levels only in formula-fed calves and increased hepatic GHR
glucocorticoid effects on the somatotropic axis. There-
binding capacity, but only in colostrum-fed calves. Colostrum
fore, one could speculate that glucocorticoids stimulate
feeding decreased IGFBP-1 and -2 plasma concentrations
the functioning of the somatotropic axis in the neonatal
and hepatic IGFBP-2 and -3 mRNA levels. In conclusion,
calf at birth.
Dexa and colostrum feeding promoted maturation of the
Several studies have recently shown that the soma-
somatotropic axis. Dexa effects partly depended on whether
totropic axis in neonatal calves works in principle and
colostrum was fed or not.
is influenced by colostrum feeding, nutrient density,
glucocorticoids; growth hormone; growth hormone receptor;
and age (13, 20, 47). Although colostral IGF-I did not
insulin-like growth factor I; insulin-like growth factor-bind-
affect systemic IGF-I levels in a significant manner,
colostrum intake increased IGF-I plasma concentra-tions and hepatic IGF-I expression in neonatal calves,whereas GH plasma concentrations behaved inconsis-
THE SOMATOTROPIC AXIS is the main endocrine system
tently with respect to colostrum feeding (13, 20, 23, 50).
regulating postnatal growth in mammals, but prenatal
Besides the large nutrient supply by colostrum feeding,
growth is rather independent of growth hormone (GH;
nonnutritive components of colostrum seem to affect
see Refs. 7 and 16). Glucocorticoids are supposed to
the IGF-I status in neonatal calves (13).
enhance the maturation of the somatotropic axis
There is minor knowledge in precocious species like
around birth in ruminants and pigs. The prepartum
cattle about effects of glucocorticoids on the somato-
cortisol surge may play an important role in initiating
tropic axis at birth and about interactions of glucocor-
the perinatal switch of the somatotropic axis from the
ticoid effects with colostrum feeding. We have found
Address for reprint requests and other correspondence: H. M.
The costs of publication of this article were defrayed in part by the
Hammon, Div. of Animal Nutrition and Physiology, Univ. of Berne,
payment of page charges. The article must therefore be hereby
Route de la Tioleyre 4, CH-1725 Posieux, Switzerland (E-mail:
marked ‘‘advertisement'' in accordance with 18 U.S.C. Section 1734
solely to indicate this fact.
0193-1849/03 $5.00 Copyright 2003 the American Physiological Society
DEXAMETHASONE AND SOMATOTROPIC AXIS IN NEONATAL CALVES
high endogenous cortisol levels around birth in calves
bottle two times daily. To ensure that all calves received the
(21, 42). Furthermore, glucocorticoids influenced the
same amounts of formula or colostrum, calves with reduced
somatotropic axis in newborn sheep and pigs (10, 30).
appetite were tube fed their planned amounts of formula or
On the basis of these premises, we administered Dexa
colostrum. Calves received their first meal at 3.1 ⫾ 0.04 h
to colostrum- and formula-fed neonatal calves, starting
(GrFD⫺), 2.2 ⫾ 0.02 h (GrFD⫹), 2.4 ⫾ 0.03 h (GrCD⫺), and3.4 ⫾ 0.04 h (GrCD⫹) after birth. The following feedings were
the treatment immediately after birth to simulate a
at 8, 24, and 32 h after the first feeding. From day 3 on, calves
high glucocorticoid status. The objective of this study
were fed daily at 0800 and at 1600.
was to clarify the effects of glucocorticoids on the so-
Colostrum was from cows of the Federal Research Station
matotropic axis and whether these effects depend on
for Animal Production. Cows were milked two times daily,
feeding colostrum or milk-based formulas that con-
and the colostrums of milkings 1 (day 1), 3 (day 2), and 5 (day
tained nutrients in similar amounts to colostrum, but
3) after parturition were stored separately in plastic bottles
much fewer growth-promoting substances like hor-
at ⫺20°C. Individual pools of milkings 1, 3, and 5 were
mones and growth factors (3, 42). We have tested the
prepared at the beginning of the study and then stored in
hypothesis that Dexa influences the maturation of the
plastic bottles at ⫺20°C until used. Before feeding, colostrum
somatotropic axis in neonatal calves and that Dexa
was warmed to 40°C and then fed immediately.
effects are modified by colostrum feeding. Therefore,
Three formulas for days 1, 2, and 3 were created that
contained nutrients (protein, fat, lactose) in comparable
we have measured various components of the somato-
amounts as colostrum milkings 1, 3, and 5 after parturition
tropic axis in blood and mRNA concentrations in liver
and were fed on days 1, 2, and 3 after birth. Formulas were
as well as GHR binding sites in liver.
produced by UFA (Sursee, Switzerland) and consisted ofcalcium-caseinate (Emmi Milch, Lucerne, Switzerland), lact-
MATERIALS AND METHODS
albumin (Emmi Milch), milk fat (double cream; Institut Ag-
Animals, husbandry, feeding, and experimental proce-
ricole de l'Etat de Fribourg, Grangeneuve, Switzerland), and
dures. The experimental procedures were approved by the
a vitamin and mineral premix (Provimi, Cossonay-Gare,
Cantonal Committee for the Permission of Animal Experi-
Switzerland). The three formulas were dissolved by adding
mentation (Granges-Paccot, Canton of Fribourg, Switzer-
water and were stored in plastic bottles at ⫺20°C until used.
land) and followed the actual law of animal protection. They
Before feeding, formulas were warmed to 40°C and then fed
were supervised by the Federal Veterinary Administration.
immediately. The milk replacer (UFA 200 natura, without
Twenty-eight male calves (11 Holstein-Friesian, 12 Simmen-
antibiotics) was prepared as a 100 g/l solution. Contents of
tal ⫻ Red Holstein, 4 Brown Swiss, and 1 Red Holstein ⫻
different colostrum milkings, formulas, and milk replacer are
Limousin) were studied. They were born at the Federal
shown in Table 2.
Research Station for Animal Production (Posieux, Switzer-
To protect against infections, all calves were subcutane-
land) or at neighboring farms. Calves were born spontane-
ously injected with 2 g of a bovine colostral immunoglobulin
ously and were separated immediately after birth from their
preparation (Gammaserin; Gra¨ub, Berne, Switzerland) be-
dams and held on straw in boxes for 5 days.
fore the first feed intake. Additionally, all calves were fed
Four treatment groups were created randomly (GrFD⫺,
chicken egg-derived immunoglobulins containing high-anti-
GrFD⫹, GrCD⫺, GrCD⫹), each consisting of seven calves
body titers against rotavirus and pathogenic Escherichia coli
(Table 1). Calves of GrCD⫺ and GrCD⫹ received colostrum of
type K 99 (Globigen 88; kindly donated by Lohmann Animal
milkings 1, 3, and 5 (days 1, 2, and 3 after parturition,
Health). Fed amounts were 10 g (day 1), 8 g (day 2), 6 g (day
respectively) on the first 3 days. Calves of GrFD⫺ and GrFD⫹
3), and 4 g (day 4)/meal. On days 1, 2, and 3, calves were
were fed three different milk-based formulas for days 1, 2,
subcutaneously injected antibiotics (25 mg Enrofloxacin/10
and 3 that represented colostral milkings 1, 3, and 5, respec-
kg body wt; 5% Baytril; Bayer, Leverkusen, Germany). To
tively. Calves of GrCD⫹ and GrFD⫹ were treated with 30
avoid different treatment protocols, drugs were given to both
g䡠kg body wt⫺1䡠day⫺1 Dexa (Dexa TAD; Lohmann Animal
formula- and colostrum-fed calves.
Health status. The health status was evaluated daily based
grouped with the goal to obtain similar body weight and
on the following clinical traits: rectal temperature, heart
breed distributions in all four groups. Calves were fed by
rate, respiratory rate, behavior, nasal discharge, respiratory
Table 1. Experimental groups
g䡠kg body wt⫺1䡠day⫺1
Days 1–4
Twenty-eight calves were randomly grouped and were fed either colostrum (GrCD⫺ and GrCD⫹) or formula (GrFD⫺ and GrFD⫹). In each
feeding group, one-half of the calves were treated with dexamethasone (Dexa) (GrCD⫹ and GrFD⫹). With Dexa treatment, calves weretreated two times daily at feeding times; dose of DEXA was chosen according to previous studies in neonatal calves (14). Day 1, colostrumand formula were fed in amounts of 6% of body wt; Day 2, colostrum and formula were fed in amounts of 8% of body wt; Days 3 and 4,colostrum and formula were fed in amounts of 10% of body wt.
AJP-Endocrinol Metab • VOL 285 • AUGUST 2003 • www.ajpendo.org
DEXAMETHASONE AND SOMATOTROPIC AXIS IN NEONATAL CALVES
Table 2. Composition of colostrum milkings, formula, and milk replacer fed to neonatal calves
Gross energy, MJ/kg
(MJ/kg dry matter)
Crude protein, g/kg
(g/kg dry matter)
Crude lipids, g/kg
(g/kg dry matter)
Nitrogen free extracts, g/kg
(g/kg dry matter)
Crude ashes, g/kg
(g/kg dry matter)
Formula (per kg) was composed of calcium-caseinate (86, 208, and 282 g on days 1, 2, and 3, respectively), lactalbumin (576, 245, and 80
g on days 1, 2, and 3, respectively), milk fat (267, 262, and 353 g double cream on days 1, 2, and 3, respectively), lactose (57, 271, and 271g on days 1, 2, and 3, respectively), and a mineral premix (14 g on days 1–3). The mineral premix (per kg) contained calcium (186 g),magnesium (224 g), sodium (31 g), phosphorus (92 g), chlorine (48 g), iron (12 g), manganese (8.1 mg), copper (1.6 mg), zinc (7.8 mg), iodine(0.03 mg), cobalt (0.02 mg), and selenium (0.02 mg). Milk replacer was fed as 10% solution (wt/vol) and was composed (per kg) of skim milkpowder (550 g), whey (40 g), corn-derived products (dextrose, glucose, oat cream, starch; 172 g), tallow (145 g), lard (44 g/kg), lecithin (asemulgator; 19 g), calcium (12 g), phosphorus (7.5 g), magnesium (1.6 g), sodium (4.9 g), zinc (80 mg), manganese (60 mg), iron (20 mg), copper(8 mg), iodine (3 mg), selenium (0.5 mg), cobalt (0.5 mg), vitamin A (26.25 mol retinol equivalent/kg), cholecalciferol (195 nmol/kg), vitaminE (360 mol ␣-tocopherol/kg;), thiamine (57 mol/kg), riboflavin (21 mol/kg), vitamin B6 (59 mol/kg), and vitamin B12 (37 nmol/kg). Milkreplacer included 30 g lactose/kg milk (320 g/kg dry matter). NM, not measurable (below detection limit). ND, not determined.
sounds, appetite, fecal consistency, and navel adspection.
and GHR mRNA, were recently described in detail (18, 40).
Body weight was determined on days 1 and 5 of life.
RT-PCR quantification was performed with the LightCycler
Blood samples. Blood samples were taken from the jugular
system (Roche Molecular Biochemicals, Rotkreuz, Switzer-
vein with evacuated tubes on days 1, 2, and 5 and with a
land), using the software package 3.3 (Roche Molecular Bio-
catheter on day 4. Tubes containing dipotassium-EDTA (1.8
chemicals). Absolute quantification was based on external
g/l blood) were used for the determination of pre- and post-
recombinant DNA standards (40) and was related to 18S
prandial values of IGF-I and IGF-binding protein (IGFBP)-1
RNA to compensate for RNA loading differences. Values were
at 0, 1, 2, 4, and 8 h after the 1st, 3rd, and 7th feeding and on
expressed on a molar basis.
day 5 and for the determination of preprandial values of
For 125I-GH-binding studies, liver samples were thawed
IGFBP-2 and IGFBP-3 on days 1 and 4, respectively. The
and transferred to receptor buffer (in mM: 50 Tris 䡠 HCl, 6
same tubes were used to measure plasma concentrations of
MgCl2, and 1 EGTA; pH 7.4; 4°C). The tissue was homoge-
GH in pre- and postprandial samples on days 1 and 2, in 25
nized four times for 20 s at low speed (8,000 U/min) with an
samples on day 4 taken before (0 h) and every 20 min after
Ultra-Turrax homogenizer (T25; Janke & Kunkel, Staufen,
the morning feeding for 8 h for the evaluation of secretory
Germany). The homogenate was centrifuged at 800 g for 10
patterns, and on day 5. Tubes were put on crushed ice and
min, and the supernatant was centrifuged at 10,000 g for 10
centrifuged at 1,000 g for 20 min. Supernatants were sepa-
min; thereafter, the resulting supernatant was centrifuged
rated into aliquots and stored at ⫺20°C.
again at 100,000 g for 1 h (22). The remaining pellet was
Blood analyses. Plasma GH, IGF-I, and IGFBP-1 concen-
resuspended in ice-cold buffer (as above) by a motor-driven
trations were measured by RIA, as described previously (20,
glass-Teflon homogenizer and stored at ⫺80°C until assayed.
26). Plasma concentrations of IGFBP-2 and IGFBP-3 were
The protein concentration was determined using a kit (BCA
measured by ligand blots using 125I-labeled IGF-I. The pro-
Protein Assay Reagent; Pierce, Rockford, IL), and then the
cedure was described recently (20, 23).
membrane suspensions were adjusted to a final protein con-
Analyses in liver. Calves were slaughtered on day 5 of life,
centration of 4 mg/ml. For receptor measurements, mem-
and liver samples were put either in liquid nitrogen (GHR
branes were suspended in incubation buffer (as above). Lin-
binding studies) or in TRIzol (GIBCO-BRL, Basel, Switzer-
earity of binding dependent on protein concentration was
land) and then frozen in liquid nitrogen. Liver samples were
tested, and the final protein concentration in assays was
stored at ⫺80°C until analyzed for mRNA of IGF-I, IGF-II,
within the linear range. Binding of 125I-recombinant bovine
IGFBP-2, IGFBP-3, and GHR and for GHR binding sites.
GH (rbGH) was measured as previously described (1, 22).
For mRNA measurements, total RNA was extracted using
The rbGH was from Monsanto (St. Louis, MO), and prolactin
TRIzol reagent (GIBCO-BRL) and resuspended in RNase-
was from the United States Department of Agriculture Ani-
free water that was treated with diethyl pyrocarbonate
mal Hormone Program (Dr. D. Bolt, Beltsville, MD). Compet-
(Sigma-Aldrich, Deisendorf, Germany). RNA integrity and
itive binding studies for the characterization of 125I-rbGH
purity were tested by measurement of optical density and by
binding sites were performed in pooled membrane suspen-
electrophoresis using ethidium bromide staining. Total RNA
sions with 0.35 ng of the radiolabeled ligand and increasing
was reverse transcribed into cDNA with hexamer primers
concentrations of unlabeled rbGH and prolactin. For the
(Pharmacia Biotech, Buckinghamshire, UK), as described
determination of the number of 125I-rbGH binding sites, the
previously (40). Materials and procedures for RT-PCR, in-
radiolabeled rbGH (0.35 ng) was incubated with increasing
cluding primers for bovine IGF-I, IGF-II, IGFBP-2, IGFBP-3,
concentrations of the unlabeled rbGH (1, 22).
AJP-Endocrinol Metab • VOL 285 • AUGUST 2003 • www.ajpendo.org
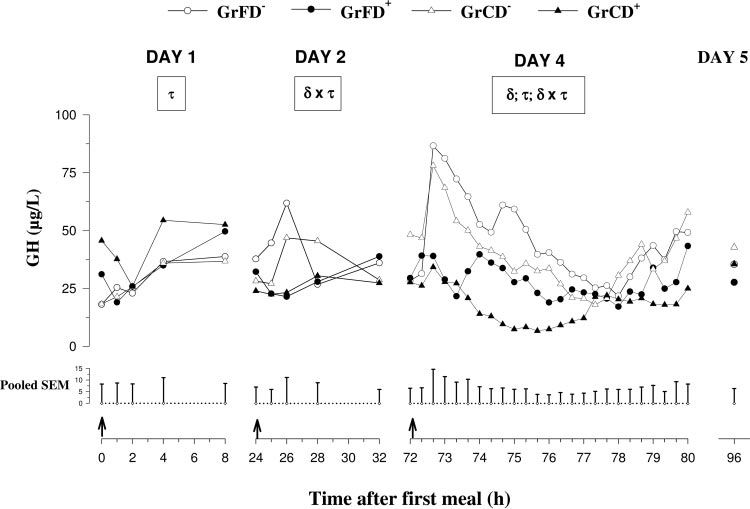
DEXAMETHASONE AND SOMATOTROPIC AXIS IN NEONATAL CALVES
Analyses in formula, colostrum, and milk replacer. Sam-
the general linear model (GLM) and differences were local-
ples of formulas 1, 2, and 3 and samples of the individual
ized by Bonferroni t-test (P ⬍ 0.05; see Ref. 45).
pools of colostrum of milkings 1, 3, and 5 were lyophilized to
Concentrations of mRNA as well as number (Bmax) and
determine dry matter, crude protein (by the Kjeldahl
50% inhibition (IC50) of GH binding sites were evaluated
method), crude fat (by the Berntrop method), and ashes (after
using the GLM (45) with Dexa treatment and feeding as
combustion at 550°C) using standard procedures at the Swiss
main effects. The Dexa ⫻ feeding interaction was included in
Federal Research Station for Animal Production. Contents of
the model. Dexa treatment and feeding effects were localized
nitrogen-free extracts and gross energy (based on energy
by the Bonferroni t-test (P ⬍ 0.05).
equivalents of 36.6, 17.0, and 24.2 MJ/kg fat, nitrogen-freeextracts, and crude protein) were calculated. Information on
contents of the milk replacer was given by the producer.
Feed intake, body weight, and health status. Feed
Concentrations of insulin and IGF-I in formula and co-
intake was the same in all four groups. The mean body
lostrum were analyzed as described by Hammon and Blum
weights of all four groups before the first meal (46.7 ⫾
Statistical procedures. Values of body weight, blood traits,
3.8 kg) were the same and did not change until day 5
mRNA concentrations, and GH binding sites were expressed
(46.5 ⫾ 3.8 kg). There were no differences in health
as means ⫾ SE or pooled SE. For plasma concentrations of
traits among the different groups (data not shown).
GH, IGF-I, and IGFBP-1, areas under the concentration
However, two calves of GrFD⫺ and GrFD⫹ had loose
curves were computed for each day as measures of mean
feces for 2 days.
concentrations between 0 and 8 h. The ratios of GH to IGF-I
Blood hormone concentrations. Preprandial plasma
plasma concentrations were calculated as means for describ-
GH concentrations (Fig. 1) did not change from day 1 to
ing the maturation of the GH-IGF-I system, as defined by
day 5 but were higher (P ⬍ 0.05) before the first feed
Gluckman et al. (19). Data were evaluated using the RAN-
intake in Dexa-treated than in nontreated calves. Con-
DOM and REPEATED methods of the MIXED procedure
centrations of GH on day 1 changed with time in an
(45). Separate models were applied for the calculation of bodyweight as well as preprandial and mean concentrations of
inconsistent manner but on day 2 remained low in
GH, IGF-I, and IGFBPs during the whole experimental pe-
Dexa-treated groups and transiently increased (P ⬍
riod and for the calculation of postprandial effects on days 1,
0.1) after feed intake in nontreated groups. On day 4,
2, and 4, respectively. Dexa treatment, feeding, and time
concentrations postprandially increased (P ⬍ 0.01) in
were used as fixed effects, and the individual calves were
nontreated groups, whereas GH concentrations re-
used as random effects. For the evaluation of differences in
mained low in Dexa-treated groups. Mean and basal
Dexa responses with regard to different feeding and for the
concentrations as well as amplitudes of secretory peaks
evaluation of differences in the time pattern within Dexa or
were lower (P ⬍ 0.05) in Dexa-treated than in non-
feeding groups, interactions (Dexa ⫻ feeding; Dexa ⫻ time;
treated groups.
feeding ⫻ time) were included in the model. Treatment,
Preprandial plasma concentrations of IGF-I (Fig. 2)
feeding, and time differences were localized by the Bonfer-roni t-test (P ⬍ 0.05). Episodic secretion of GH on day 4
decreased (P ⬍ 0.01) in nontreated calves from day 1 to
(mean concentrations, basal concentrations, peak ampli-
day 5. Mean concentrations remained unchanged in
tudes, and peak frequencies) was analyzed according to Mer-
Dexa-treated calves from day 1 to day 2 but decreased
riam and Wachter (36). Group differences were analyzed by
(P ⬍ 0.01 in GrFD⫹; P ⬍ 0.1 in GrCD⫹) from day 2 to
Fig. 1. Plasma pre- and postprandialgrowth hormone (GH) concentrationson days 1 and 2, secretory patternsmeasured during 8 h every 20 min af-ter feed intake on day 4, and prepran-dial concentrations on day 5 in neona-tal calves fed with formula or co-lostrum
GrCD⫺ (‚)] and with Dexa injections[GrFD⫹ (F) and GrCD⫹ (Œ)]. On day 4,all calves were fed with a milk re-placer. Values are presented as means,and pooled SE is shown at bottom (n ⫽7). ␦, Dexa effect (P ⬍ 0.05); , feedingeffect (P ⬍ 0.05); , time effect (P ⬍0.05); ␦ ⫻ , Dexa ⫻ feeding interac-tion (P ⬍ 0.05); ␦ ⫻ , Dexa ⫻ timeinteraction (P ⬍ 0.05); ⫻ , feeding ⫻time interaction (P ⬍ 0.05). Arrowsmark times of feed intake.
AJP-Endocrinol Metab • VOL 285 • AUGUST 2003 • www.ajpendo.org
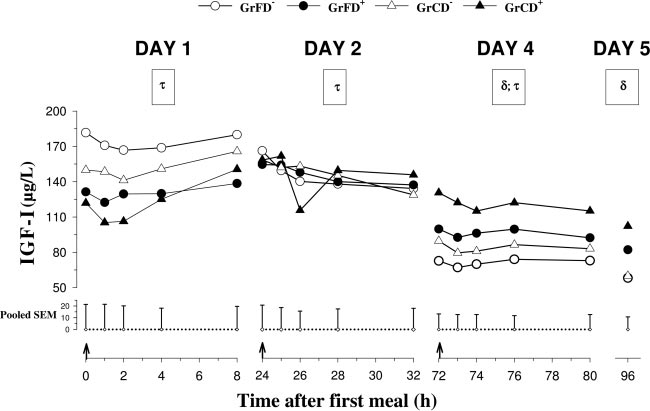
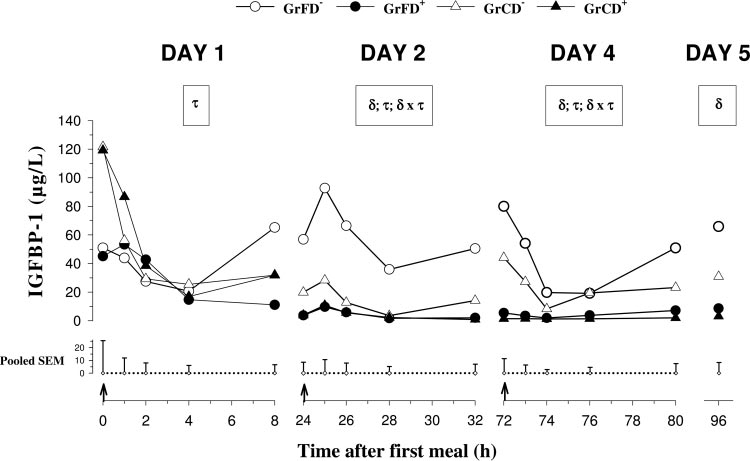
DEXAMETHASONE AND SOMATOTROPIC AXIS IN NEONATAL CALVES
Fig. 2. Plasma pre- and postprandial IGF-I concen-trations on days 1, 2, and 4 and preprandial concen-trations on day 5 in neonatal calves fed with formulaor colostrum without Dexa injections [GrFD⫺ (E)and GrCD⫺ (‚)] and with Dexa injections [GrFD⫹ (F)and GrCD⫹ (Œ)]. On day 4, all calves were fed with amilk replacer. For further details see legend toFig. 1.
day 4. Postprandial concentrations changed (P ⬍ 0.05)
after feed intake in colostrum-fed calves and responded
with time after feed intake on days 1, 2, and 4, de-
(P ⬍ 0.05) to feed intake on days 2 and 4 in nontreated
creased in GrFD⫺ (P ⬍ 0.01) on day 2, and decreased
calves. Preprandial IGFBP-1 concentrations on day 1
(P ⬍ 0.05) in colostrum-fed groups on day 4. Prepran-
were higher (P ⬍ 0.05) in colostrum-fed than in for-
dial IGF-I concentrations on day 1 were lower (P ⬍
mula-fed calves. IGFBP-1 concentrations from day 2 to
0.05) in Dexa-treated than in nontreated calves. Dexa
day 5 were lower (P ⬍ 0.05) in Dexa-treated than in
treatment increased (P ⬍ 0.05) IGF-I concentrations
nontreated calves. On days 2 and 5, IGFBP-1 concen-
compared with nontreated calves on days 4 and 5. The
trations tended to be higher in GrFD⫺ than in GrCD⫺.
ratios of mean GH to mean IGF-I plasma concentra-
Plasma concentrations of IGFBP-2 increased (P ⬍
tions on day 4 were lower (P ⬍ 0.001) in Dexa-treated
0.05) in GrFD⫺ and decreased (P ⬍ 0.05) in GrCD⫹
than in nontreated calves and tended to be lower (P ⬍
from day 1 to day 4 (Table 3). Plasma concentrations of
0.1) in colostrum-fed than in formula-fed calves (ratios
IGFBP-2 on day 4 were lower (P ⬍ 0.05) in Dexa-
were 0.68 ⫾ 0.1, 0.3 ⫾ 0.03, 0.53 ⫾ 0.1, and 0.14 ⫾
treated than in nontreated calves and were lower in
0.004 for GrFD⫺, GrFD⫹, GrCD⫺, and GrCD⫹, respec-
colostrum-fed than in formula-fed calves, and the
Dexa-induced reduction was greater (P ⬍ 0.01) in for-
Preprandial plasma concentrations of IGFBP-1 (Fig.
mula-fed than in colostrum-fed calves. Plasma concen-
3) decreased in GrFD⫹, GrCD⫺, and GrCD⫹ (P ⬍ 0.05)
trations of IGFBP-3 decreased (P ⬍ 0.05) in GrFD⫺
from day 1 to day 2 and remained unchanged until day
from day 1 to day 4 (Table 3). There were no differences
5. Plasma concentrations decreased on day 1 (P ⬍ 0.05)
concerning Dexa treatment or feeding.
Fig. 3. Plasma pre- and postprandialIGF-binding protein (IGFBP)-1 con-centrations on days 1, 2, and 4 andpreprandial concentrations on day 5 inneonatal calves fed with formula orcolostrum
[GrFD⫺ (E) and GrCD⫺ (‚)] and withDexa injections [GrFD⫹ (F) and GrCD⫹(Œ)]. On day 4, all calves were fed witha milk replacer. For further details seelegend to Fig. 1.
AJP-Endocrinol Metab • VOL 285 • AUGUST 2003 • www.ajpendo.org
DEXAMETHASONE AND SOMATOTROPIC AXIS IN NEONATAL CALVES
Table 3. Plasma concentrations of IGFBP-2 and IGFBP-3 in newborn calves fed with formula or colostrumwith (GrFD⫹, GrCD⫹) or without Dexa injections (GrFD⫺, GrCD⫺)
ANOVA (P values)
Values are means; n ⫽ 7 animals/group. Units are optical density ⫻ 103. IGFBP, IGF-binding protein; SE, pooled standard error; ␦, Dexa
effect; , feeding effect; ␦⫻, Dexa ⫻ feeding interaction. NS, statistically not significant. * P ⬍ 0.05 and † P ⬍ 0.01.
Hepatic mRNA concentrations on day 5 of life. Ex-
However, concentrations of biologically active factors
pression of IGF-I tended to be higher (P ⬍ 0.1) in
like IGF-I or insulin were much lower in the three
Dexa-treated than in nontreated calves and was higher
formulas than in colostrum and possibly affected neo-
(P ⬍ 0.05) in GrFD⫹ than in GrFD⫺ and in GrCD⫹
natal development because receptors for IGF-I and
(Table 4). Expression of IGF-II tended to be higher (P ⬍
insulin are present in the gut mucosa of neonatal
0.1) in formula-fed than in colostrum-fed calves. Dexa
calves (18, 22). Body weight remained stable during
treatment resulted (P ⬍ 0.1) in different responses to
the experimental period, and Dexa treatment or differ-
feed intake in formula-fed and colostrum-fed calves.
ent feeding did not affect growth performance, yet the
Expression of IGFBP-2 and IGFBP-3 was higher (P ⬍
experimental period of 5 days was too short to get
0.001 and P ⬍ 0.01) in formula-fed than in colostrum-
reliable data on effects of Dexa on growth performance,
fed calves. Calves treated with Dexa showed higher
as was the case in 18-day-old pigs (10).
expression (P ⬍ 0.01) of GHR than nontreated calves.
Feeding and treatment effects on GH and GHR.
Hepatic GH binding sites on day 5 of life. In 125I-GH
Plasma GH concentrations in our study were not influ-
binding studies (Fig. 4A), IC50 of unlabeled rbGH was
enced by different feeding but were reduced by Dexa
4.1 ⫻ 10⫺10 M, and IC50 of prolactin was 5.4 ⫻ 10⫺6 M.
treatment. Plasma GH concentrations were barely reg-
Dexa treatment resulted in different effects on Bmax in
ulated by feeding in neonatal calves (20, 23, 42),
formula-fed and colostrum-fed calves (P ⬍ 0.1 for
whereas they were influenced by feeding intensity in
Dexa ⫻ feeding interaction), and Bmax tended to be
older cattle (7, 43). Because nutrient intake was the
higher (P ⬍ 0.1) in GrCD⫹ than in GrCD⫺ (Fig. 4B).
same in the present study, nonnutritive factors such as
IC50 of GH binding sites were similar in different
IGFs, IGFBPs, and lactoferrin, which are present in
high amounts in colostrum (3) but not in the formulas,obviously did not influence GH secretion. In cattle,
plasma GH concentrations decrease during ontoge-
Feed intake, body weight, and health status. De-
netic development (8, 14). Because glucocorticoids are
creases in dry matter, gross energy, crude protein,
important for neonatal maturation (7), reduced GH
crude fat, crude ashes, insulin, and IGF-I concentra-
concentrations after Dexa treatment in our study
tions but an increase in nitrogen-free extracts (mainly
might indicate enhanced maturation of the somato-
of lactose) in colostrum of milkings 1, 3, and 5 fed to
tropic axis, as shown in neonatal pigs (10). On the
neonatal calves have also been observed in earlier
other hand, cortisol treatment in 1-mo-old calves did
studies (42). Because formulas of days 1, 2, and 3
not affect plasma GH concentrations (15). Because
contained similar amounts of nutrients as the co-
glucocorticoids have stimulatory and inhibitory effects
lostrum of milkings 1, 3, and 5 and because on day 4 all
on pituitary GH production and release (38), effects of
calves received the same milk replacer, nutrient in-
glucocorticoids on GH secretion may depend on the
takes were comparable in different feeding groups.
developmental status. In addition, reduced GH concen-
Table 4. Expression of IGF-I, IGF-II, IGFBP-2, IGFBP-3, and GHR on day 5 of life in liver of newborn calvesfed with formula or colostrum with (GrFD⫹, GrCD⫹) or without Dexa injections (GrFD⫺, GrCD⫺)
ANOVA (P Values)
IGF-I (⫻10⫺6)
IGF-II (⫻10⫺3)
IGFBP-2 (⫻10⫺6)
IGFBP-3 (⫻10⫺6)
Values are means; n ⫽ 7 animals/group. Units are fmol/g total RNA. GHR, growth hormone receptor NS, statistically not significant. * P ⬍
0.1, † P ⬍ 0.01, and ‡ P ⬍ 0.001. Means with different capital letter superscripts (A, B) are significantly different (P ⬍ 0.05) within Dexatreatment; means with different small letter superscripts (a, b) are significantly different (P ⬍ 0.05) within feeding.
AJP-Endocrinol Metab • VOL 285 • AUGUST 2003 • www.ajpendo.org
DEXAMETHASONE AND SOMATOTROPIC AXIS IN NEONATAL CALVES
nied by colostrum feeding, supports maturation of thesomatotropic axis by stimulating hepatic GHR synthe-sis. Possibly, glucose might have caused the increase ofGHR expression because glucose stimulated GHRmRNA in porcine hepatocytes (4) and because we foundthe highest glucose plasma concentrations in GrCD⫹(Sauter SN, Blum JW, and Hammon HM, unpublishedobservation). Higher hepatic GH binding sites are animportant basis for enhanced IGF-I responses to GH,and the response usually increases with age (8). Theeffects of Dexa treatment on GHR correspond to theview that parturition-associated mechanisms (such ashigh cortisol levels) are necessary for the postnatalincrease of hepatic GH binding sites (6). Obviously,Dexa administration mimics the cortisol surge at birthand causes this postnatal hepatic GHR stimulation.
Because the stimulation of GHR expression by Dexawas observed in both feeding groups but stimulation ofGH binding by Dexa was only noticed in colostrum-fedcalves, feeding of colostrum may regulate posttran-scriptional GHR synthesis in Dexa-treated calves.
Feeding and Dexa effects on IGF-I expression and
plasma concentrations. Although IGF-I plasma concen-trations were numerically higher in colostrum-fed thanin formula-fed calves and concentrations of hepaticIGF-I mRNA tended to increase in GrCD⫺ comparedwith GrFD⫺, colostrum effects on neonatal IGF-I levelswere not significant in the present study. These find-ings are contrary to our previous results (13, 20), butcalves of those studies differed in age of the calvesand/or feeding protocol.
Treatment with Dexa increased IGF-I plasma con-
centrations in this study, although glucocorticoids areknown to inhibit postnatal growth in association with
Fig. 4. 125I-labeled GH binding to 4 mg of pooled membrane suspen-sions dependent on increasing concentrations of unlabeled GH (F)
reduced IGF-I plasma concentrations (9, 48, 49). How-
and prolactin (PRL; ‚; A) and the number of 125I-GH binding sites in
ever, our data correspond to findings in neonatal pigs
the liver of neonatal calves fed formula or colostrum without Dexa
(10) and growing bulls (43) treated with Dexa. How-
injections (GrFD⫺ and GrCD⫺) and with Dexa injections (GrFD⫹ and
ever, Coxam et al. (15) did not find an effect of cortisol
GrCD⫹) on day 5 of life (B).
on plasma IGF-I concentrations in calves, and Dexadecreased plasma IGF-I concentrations in cows and
trations in Dexa-treated calves possibly resulted from
finishing calves (2, 33). Therefore, effects of glucocorti-
elevated IGF-I concentrations because of negative
coids on IGF-I plasma concentrations are variable and
feedback mechanisms of IGF-I on pituitary GH secre-
may depend on treatment-dose (34) and age (9). In any
tion (38). We have demonstrated a negative feedback
case, plasma IGF-I concentrations increase during
mechanism in neonatal calves resulting from Long-R3-
postnatal development in cattle (7, 28, 44). Therefore,
elevated IGF-I concentrations in Dexa-treated calves,
Hepatic GHR mRNA concentrations (especially the
lower GH-to-IGF-I ratios in Dexa-treated calves, and
GHR 1A variant) and GHR binding in cattle are low at
the trend of lower GH-to-IGF-I ratios in colostrum-fed
birth and increase with age, indicating ontogenetic
than in formula-fed calves indicate enhanced matura-
maturation of the somatotropic axis (1, 7, 31, 43). GHR-
tion of the somatotropic axis, as defined by Gluckman
binding studies revealed an almost fivefold increase of
et al. (19).
Bmax in bulls (450 kg body wt) compared with neonatal
Increased IGF-I plasma concentrations in Dexa-
calves (Zbinden Y, Blum JW, and Hammon HM, un-
treated calves might result from different clearance
published data). In our study, colostrum intake did not
rates of IGF-I. Our study has demonstrated reduced
influence GHR expression and GH binding in liver, but
plasma concentrations of IGFBP-1 and -2 in Dexa-
Dexa treatment increased hepatic GHR expression.
treated calves. These binding proteins are able to leave
The greatest GH binding was seen in calves fed co-
the blood circulation together with IGF-I. Because we
lostrum and treated by Dexa. Glucocorticoids stimu-
found no differences in plasma concentrations of
lated hepatic GHR mRNA in fetal sheep (30), neonatal
IGFBP-3, which (together with its acid-labile subunit)
pig (10), rabbit (24), and porcine (5) hepatocytes. Our
retains most IGF-I in circulation (25), and because the
findings indicate that Dexa, especially when accompa-
ratios of small IGFBPs to IGFBP-3 were lower in
AJP-Endocrinol Metab • VOL 285 • AUGUST 2003 • www.ajpendo.org
DEXAMETHASONE AND SOMATOTROPIC AXIS IN NEONATAL CALVES
Dexa-treated calves, clearance of IGF-I from the circu-
plasma concentrations in Dexa-treated calves. We did
lation might have been reduced in Dexa-treated calves.
not measure hepatic IGFBP-1 gene expression in this
Furthermore, insulin, which was elevated in Dexa-
study, but plasma concentrations of IGFBP-1 were
treated calves (Sauter SN, Blum JW, and Hammon
regulated directly at the transcriptional level (12), and
HM, unpublished observation) and enhances plasma
insulin overcame stimulatory effects of glucocorticoids
IGF-I concentrations in cows (35), might have caused
on hepatic IGFBP-1 mRNA levels (37, 39). Further-
increased plasma IGF-I concentrations.
more, insulin suppressed IGFBP-2 plasma concentra-
Hepatic production of IGF-I mainly determines cir-
tions in cows (35), which is in accord with the present
culating IGF-I concentrations (9, 13, 25). Hepatic IGF-I
study. However, Dexa treatment did not influence he-
expression increased after Dexa treatment in GrFD⫹
patic expression of IGFBP-2, indicating that it did not
but decreased in GrCD⫹. Thus, in formula-fed calves,
regulate IGFBP-2 at the transcriptional level.
there was a positive association of IGF-I mRNA con-
In addition, plasma IGFBP-2 concentrations in neo-
natal calves are regulated by the amount and time
whereas there was a negative association in colostrum-
point of colostrum intake (20, 23). In our study, de-
fed calves. An inverse behavior of IGF-I plasma con-
creased IGFBP-2 plasma concentrations in colostrum-
centrations and hepatic expression was also seen in
fed calves were associated with reduced hepatic
neonatal Dexa-treated and colostrum-fed pigs (10). Dif-
IGFBP-2 mRNA levels. Because nutrient intake was
ferent Dexa effects on IGF-I expression in formula- and
the same in formula- and colostrum-fed calves, other
colostrum-fed calves were surprising, because we have
factors than nutrient density were responsible for re-
previously shown a significant relationship between
duced IGFBP-2 levels. Overall, decreasing levels of
hepatic IGF-I mRNA and plasma concentrations in
IGFBP-1 and IGFBP-2 induced by Dexa treatment
8-day-old calves (13). One could speculate that colos-
and/or colostrum feeding in the present study support
tral IGF-I was intestinally absorbed and that Dexa
the hypothesis of an accelerated maturation of the soma-
treatment might have stimulated the absorption of
totropic axis, as seen in postnatal development (7).
colostral IGF-I, thus resulting in reduced hepatic IGF-I
Furthermore, we found reduced hepatic IGFBP-3
expression in colostrum-fed calves because of feedback
mRNA levels after colostrum feeding, but this was not
inhibition (20, 38). However, there was no evidence for
associated with reduced plasma concentrations. Con-
increased absorption of macromolecules like IgG in
centrations of IGFBP-3 mRNA did not go along with
Dexa-treated calves (Sauter SN, Blum JW, and Ham-
hepatic IGF-I mRNA. Because effects of GH on the
mon HM, unpublished observation), and we could not
hepatic IGF system were weak, expression of IGFBP-3
demonstrate significant absorption of IGF-I in neona-
was likely not regulated by GH and IGF-I as it is in the
tal calves (20, 50), although receptors for IGF-I are
case of growing and adult animals (7, 12, 41). Previous
present in intestinal mucosa (18, 22). Different feeding
findings support lacking colostral effects on IGFBP-3
may influence hepatic IGF-I mRNA stability after
plasma concentrations (20). Surprisingly, hepatic ex-
Dexa treatment and may regulate IGF-I production atthe posttranscriptional level. Dexa depressed hepatic
pression and plasma concentrations of IGFBP-3 were
IGF-I mRNA levels in rats but increased IGF-I plasma
not affected by Dexa treatment. We expected increased
concentrations in a dose-dependent manner (32). Inter-
IGFBP-3 concentrations in Dexa-treated calves, be-
estingly, the hepatic IGF-II mRNA responses to feed-
cause IGF-I and insulin were elevated and are known
ing and Dexa treatment were comparable to IGF-I
to stimulate IGFBP-3 production (12, 27). However,
mRNA responses, pointing at similar regulatory mech-
glucocorticoids reduced IGFBP-3 plasma concentra-
anisms for hepatic IGF-I and IGF-II mRNA.
tions in finishing calves (2), and Dexa did not influence
Feeding and Dexa effects on IGFBPs. Biological ef-
hepatic IGFBP-3 mRNA in neonatal pigs (10). There-
fects of IGF-I and IGF-II greatly depend on the pres-
fore, IGFBP-3 levels in neonatal calves do not support
ence of IGFBPs. Plasma concentrations of IGFBP-1
the view of an accelerated maturation of the somato-
and -2 are high during fetal development and decrease
tropic axis by colostrum feeding or Dexa treatment.
after birth, whereas IGFBP-3, which (together with an
In conclusion, the ratios of GH to IGF-I plasma
acid-labile subunit) binds most of the IGF-I in blood,
concentrations in the present study were reduced by
increases in blood after birth (7, 12, 27, 41). These
Dexa treatment and by colostrum feeding. Because a
patterns were also shown in calves (46). Plasma con-
low GH-to-IGF-I ratio mirrors maturation of the soma-
centrations of IGFBP-1 and -2 were reduced by Dexa
totropic axis in neonates (19), both colostrum intake
treatment in the present study. Effects of glucocorti-
and Dexa treatment promoted maturation of the GH-
coids on IGFBP-1 plasma concentrations are variable;
IGF-I axis, particularly if they were combined. Vari-
inhibitory and stimulatory effects are reported (12, 41).
able hepatic IGF-I mRNA responses after Dexa treat-
On the other hand, glucocorticoids consistently depress
ment depended on different feeding, indicating differ-
IGFBP-2 plasma concentrations (12, 27, 41). Because
ences in IGF-I regulation. The higher number of GHR
insulin (Sauter SN, Blum JW, and Hammon HM, un-
binding in colostrum-fed and Dexa-treated calves
published observation) and IGF-I plasma concentra-
might have induced greater hepatic GH sensitivity and
tions were elevated in Dexa-treated calves and both
confirms the view of an accelerated maturation of the
factors depress IGFBP-1 levels (12, 29), insulin and/or
somatotropic axis. Furthermore, because nutrient in-
IGF-I may have caused the reduction of IGFBP-1
take was the same for all calves in the present study,
AJP-Endocrinol Metab • VOL 285 • AUGUST 2003 • www.ajpendo.org
DEXAMETHASONE AND SOMATOTROPIC AXIS IN NEONATAL CALVES
Dexa effects on the GH-IGF-I system were not influ-
15. Coxam V, Davicco MJ, Durand D, Bauchart D, Opmeer F,
enced by variable nutrient intake.
and Barlet JP. Steroid hormone may modulate hepatic somato-
medin C production in newborn calves. Biol Neonate 58: 16–23,
We thank Y. Aeby, J. Sturny, and their staff at the Swiss Federal
Research Station for Animal Production, Posieux, Switzerland, for
16. Fowden AL. Endocrine regulation of fetal growth. Reprod Fertil
putting the calves at our disposal, and E. Husman (UFA AG, Sursee,
Dev 7: 351–363, 1995.
Switzerland) for helping us develop the milk-based formula.
17. Fowden A L, Szemere J, Hughes P, Gilmour PS, and For-
This work was presented as part of a thesis of S. N. Sauter at the
head AJ. The effects of cortisol on the growth rate of the sheep
Faculty of Veterinary Medicine, University of Berne, 2002.
fetus during late gestation. J Endocrinol 151: 97–105, 1996.
18. Georgiev IP, Georgieva TM, Pfaffl M, Hammon HM, and
Blum JW. Insulin-like growth factor and insulin receptors in
intestinal mucosa of neonatal calves. J Endocrinol 176: 121–132,
This study was supported by Swiss National Science Foundation
Grant No. 32–59311.99.
19. Gluckman P D, Sizonenko SV, and Bassett NS. The transi-
tion from fetus to neonate: an endocrine perspective. Acta Pae-
diatr Suppl 428: 7–11, 1999.
20. Hammon H and Blum JW. The somatotropic axis in neonatal
1. Badinga L, Collier RJ, Thatcher WW, Wilcox CJ, Head HH,
calves can be modulated by nutrition, growth hormone, and
and Bazer FW. Ontogeny of hepatic bovine growth hormone
Long-R3-IGF-I. Am J Physiol Endocrinol Metab 273: E130–
receptors in cattle. J Anim Sci 69: 1925–1934, 1991.
2. Bertozzi C, Protetelle D, Massart S, Prandi A, Darras V,
21. Hammon H and Blum JW. Metabolic and endocrine traits of
Room G, Tassinari M, Vleurick L, Parmentier I, Haeze-
neonatal calves are influenced by feeding colostrum for different
broeck V, Decuypere E, Burny A, and Renaville R. Dexa-
methasone ester treatment alters insulin-like growth factor-I, its
durations or only milk replacer. J Nutr 128: 624–632, 1998.
binding proteins and thyroid status in finishing calves. Can J
22. Hammon HM and Blum JW. Feeding different amounts of
Anim Sci 80: 329–335, 2000.
colostrum or only milk replacer modify receptors of intestinal
3. Blum JW and Baumrucker CR. Colostral and milk insulin-
insulin-like growth factors and insulin in neonatal calves. Do-
like growth factors and related substances: mammary gland and
mest Anim Endocrinol 22: 155–168, 2002.
neonatal (intestinal and systemic) targets. Domest Anim Endo-
23. Hammon HM, Zanker IA, and Blum JW. Delayed colostrum
crinol 23: 101–110, 2002.
feeding affects IGF-I and insulin plasma concentrations in neo-
4. Brameld JM, Gilmour RS, and Buttery PJ. Glucose and
natal calves. J Dairy Sci 83: 85–92, 2000.
amino acids interact with hormones to control expression of
24. Heinrichs C, Yanovski JA, Roth AH, Yu YM, Domene´ HM,
insulin-like growth factor-I and growth hormone receptor mRNA
Yano K, Cutler B Jr, and Baron J. Dexamethasone increases
in cultured pig hepatocytes. J Nutr 129: 1298–1306, 1999.
growth hormone receptor messenger ribonucleic acid levels in
5. Brameld JM, Weller PA, Saunders JC, Buttery PJ, and
liver and growth plate. Endocrinology 135: 1113–1118, 1994.
Gilmour RS. Hormonal control of insulin-like growth factor-I
25. Jones JI and Clemmons DR. Insulin-like growth factors and
and growth hormone receptor mRNA expression by porcine
their binding proteins: biological action. Endocr Rev 16: 3–34, 1995.
hepatocytes in culture. J Endocrinol 146: 239–245, 1995.
26. Kaufhold JN, Hammon HM, Bruckmaier RM, Breier BH,
6. Breier BH, Ambler GR, Sauerwein H, Surus A, and Gluck-
and Blum JW. Postprandial metabolism and endocrine status
man PD. The induction of hepatic somatotrophic receptors after
in veal calves fed at different feeding frequencies. J Dairy Sci 83:
birth in sheep is dependent on parturition-associated mecha-
2480–2490, 2000.
nisms. J Endocrinol 141: 101–108, 1994.
27. Kelley KM, Oh Y, Gargosky SE, Gucev Z, Matsumoto T,
7. Breier BH, Oliver MH, and Gallaher BW. Regulation of
Hwa V, Ng L, Simpson DM, and Rosenfeld RG. Insulin-like
growth and metabolism during postnatal development. In: Ru-minant Physiology: Digestion, Metabolism, Growth and Repro-
growth factor-binding proteins (IGFBPs) and their regulatory
duction, edited by Cronje´ PB. New York: CABI, 2000, p. 187–
dynamics. Int J Biochem Cell Biol 28: 619–637, 1996.
28. Kerr DE, Laarveld B, Fehr MI, and Manns JG. Profiles of
8. Breier BH and Sauerwein H. Regulation of growth in rumi-
serum IGF-I concentrations in calves from birth to eighteen
nants by the somatotropic axis. In: Ruminant Physiology: Diges-
months of age and in cows throughout the lactation cycle. Can J
tion, Metabolism, Growth and Reproduction, edited by En-
Anim Sci 71: 695–705, 1991.
gelhardt WV, Leonhard-Marek S, Breves G, and Giesecke D.
29. Lee PD, Suwanichkul A, DePaolis LA, Snuggs MB, Morris
Stuttgart, Germany: Verlag, 1995, p. 451–474.
SL, and Powel DR. Insulin-like growth factor (IGF) suppres-
9. Butler AA and LeRoith D. Control of growth by the somato-
sion of IGFBP-1 production: evidence for mediation by the type I
tropic axis: growth hormone and the insulin-like growth factors
IGF receptor. Regul Pept 48: 199–206, 1993.
have related and independent roles. Annu Rev Physiol 63: 141–
30. Li J, Owens JA, Owens PC, Saunders JC, Fowden AL, and
Gilmour RS. The ontogeny of hepatic growth hormone receptor
10. Carroll JA. Dexamethasone treatment at birth enhances neo-
and insulin-like growth factor 1 gene expression in the sheep
natal growth in swine. Domest Anim Endocrinol 21: 97–109,
during late gestation: developmental regulation by cortisol. En-
docrinology 137: 1650–1657, 1996.
11. Carroll JA, Daniel JA, Keisler DH, and Matteri RL. Post-
31. Lucy MC, Boyd CK, Koenigsfeld AT, and Okamura CS.
natal function of the somatotrophic axis in pigs born naturally or
Expression of somatotropin receptor messenger ribonucleic acid
by caesarian section. Domest Anim Endorinol 19: 39–52, 2000.
in bovine tissues. J Dairy Sci 81: 1889–1895, 1998.
12. Clemmons DR. Insulin-like growth factor binding proteins and
32. Luo J and Murphy LJ. Dexamethasone inhibits growth hor-
their role in controlling IGF actions. Cytokine Growth Factor Rev
mone induction of insulin-like growth factor-I (IGF-I) messenger
8: 45–62, 1997.
13. Cordano P, Hammon HM, Morel C, Zurbriggen A, and
ribonucleic acid (mRNA) in hypophysectomized rats and reduced
Blum JW. mRNA of insulin-like growth factor (IGF) quantifica-
IGF-I mRNA abundance in the intact rat. Endocrinology 125:
tion and presence of IGF binding proteins, and receptors for
165–171, 1989.
growth hormone, IGF-I and insulin, determined by reverse tran-
33. Marciel SM, Chamberlain CS, Wettemann RP, and Spicer
scribed polymerase chain reaction, in the liver of growing and
LJ. Dexamethasone influences endocrine and ovarian function
mature male cattle. Domest Anim Endocrinol 19: 191–208, 2000.
in dairy cattle. J Dairy Sci 84: 1998–2009, 2001.
14. Coxam V, Davicco MJ, Dardillat C, Robelin J, Lefaivre J,
34. McCusker RH. Controlling insulin-like growth factor activity
Opmeer F, and Barlet JP. Regulation of growth hormone
and the modulation of insulin-like growth factor binding protein
release in fetal calves. Biol Neonate 54: 160–168, 1988.
and receptor binding. J Dairy Sci 81: 1790–1800, 1998.
AJP-Endocrinol Metab • VOL 285 • AUGUST 2003 • www.ajpendo.org
DEXAMETHASONE AND SOMATOTROPIC AXIS IN NEONATAL CALVES
35. McGuire MA, Dwyer DA, Harrell RJ, and Bauman DE.
42. Rauprich ABE, Hammon HM, and Blum JW. Effects of
Insulin regulates circulating insulin-like growth factors and
feeding colostrum and a formula with nutrient contents as co-
some of their binding proteins in lactating cows. Am J Physiol
lostrum on metabolic and endocrine traits in neonatal calves.
Endocrinol Metab 269: E723–E730, 1995.
Biol Neonate 78: 53–64, 2000.
36. Merriam GR and Wachter KW. Algorithms for the study of
43. Renaville R, Hammadi M, and Portetelle D. Role of the
episodic hormone secretion. Am J Physiol Endocrinol Metab 243:
somatotropic axis in the mammalian metabolism. Domest Anim
E310–E318, 1982.
Endocrinol 23: 351–360, 2002.
37. Miura Y, Higashi Y, Kato H, Takahashi SI, and Noguchi T.
44. Ronge H and Blum J. Insulin-like growth factor I during
Effects of dexamethasone on the production of insulin-like
growth in bulls. Reprod Nutr Dev 29: 105–111, 1989.
growth factor-I and insulin-like growth factor binding proteins
45. SAS Institute. SAS Users' Guide: Statistics. Cary, NC: SAS
in primary cultures of rat hepatocytes. Biosci Biotech Biochem
Institute, 1994.
56: 1396–1400, 1992.
46. Skaar TC, Baumrucker CR, Deaver DR, and Blum JW. Diet
¨ ller EE, Locatelli V, and Cocchi D. Neuroendocrine con-
effects and ontogeny of alterations of circulating insulin-like
trol of growth hormone secretion. Physiol Rev 79: 511–607, 1999.
growth factor binding proteins in newborn dairy calves. J Anim
39. Orlowski CC, Ooi GT, Brown DR, Yang YW, Tseng LY, and
Sci 72: 421–427, 1994.
Rechler MM. Insulin rapidly inhibits insulin-like growth fac-
47. Smith JM, Van Amburgh ME, Dı´az MC, Lucy MC, and
tor-binding protein-1 gene expression in H4-II-E rat hepatoma
Bauman DE. Effects of nutrient intake on the development of
cells. Mol Endocrinol 5: 1180–1187, 1991.
the somatotropic axis and its responsiveness to GH in Holstein
40. Pfaffl MW, Georgieva TM, Georgiev IP, Ontsouka E, Hage-
bull calves. J Anim Sci 80: 1528–1537, 2002.
leit M, and Blum JW. Real-time RT-PCR quantification of
48. Spencer GSG. Hormonal systems regulating growth. Livest
insulin-like growth factor (IGF)-1, IGF-1 receptor, IGF-2, IGF-2
Prod Sci 12: 31–46, 1985.
receptor, insulin receptor, growth hormone receptor, IGF-bind-
49. Unterman TG and Phillips LS. Glucocorticoid effects on so-
ing proteins 1, 2 and 3 in the bovine species. Domest Anim
matomedins and somatomedin inhibitors. J Clin Endocrinol
Endocrinol 22: 91–102, 2002.
Metab 61: 618–626, 1985.
41. Rajaram S, Baylink DJ, and Mohan S. Insulin-like growth
50. Vacher PY, Bestetti G, and Blum JW. Insulin-like growth
factor-binding proteins in serum and other biological fluids:
factor I absorption in the jejunum of neonatal calves. Biol Neo-
regulation and functions. Endocr Rev 18: 801–831, 1997.
nate 68: 354–367, 1995.
AJP-Endocrinol Metab • VOL 285 • AUGUST 2003 • www.ajpendo.org
Source: http://www.qpcrupdate.eu/sauter-ajpendo-2003.pdf
Application for registration FOR OFFICE USE Office date stamp PAYE Reference number SDL Reference number UIF Reference number Area code Please refer to the Guide for completion of Employer Registration application. Your registration may be delayed if you do not complete this application fully.
Mobile audio assistance in bus transportation for the blind J H Sánchez and C A Oyarzún Department of Computer Science, University of Chile, Blanco Encalada 2120, Santiago, CHILE ABSTRACT People with visual disabilities have serious difficulties when mobilizing through the city on the public transportation system. We introduce AudioTransantiago, a handheld application that allows users to plan trips and provide contextual information during the journey through the use of synthesized voices. The usability and cognitive evaluation of AudioTransantiago was performed using a prototype evaluation in order to identify and solve usability issues, ending up with an intuitive and simple interface. Finally, a cognitive impact evaluation administered during bus trips taken with the assistance of AudioTransantiago demonstrated that the software provides more autonomy and effectiveness for users' trips, improving their orientation and mobility.